-
Please check the real-time availability at the top of the product page (its located below the price). We will be fulfilling orders in the order they are received.
|
-
Orders will be shipped out within 2-3 business days as long as the masks are in stock. If there is a lead time posted, please expect to wait this long before the order can ship.
|
-
Lead time is the time between placing your order and when we can actually get it shipped. From there, the delivery time will depend on the shipping speed you choose on your order.
|
-
Lead time + estimated delivery time from California to your destination (based on your chosen FedEx shipping speed).
|
|
-
THEY ARE NOT, these masks are non-sterile and non-medical. They should NOT be used for surgical procedures. These masks are FDA certified and approved for general and industrial use. For the purposes of the current global pandemic these masks offer good general protection.
|
|
-
Our manufacturers are registered with the FDA for the production of our masks. The FDA does not issue official certificates, but we do have our manufacturers FDA registration information and the specific device listing number for the masks we offer.
|
|
-
At this time, unfortunately, we are not accepting international orders (outside of the USA) for our mask or other lab supplies. We are focusing on providing these supplies to our US customers who are in the most need right now. Sorry about the inconvenience.
|
-
For best results, we recommend that you Log In or Create an Account on our website first. If this step is not completed first, you will be redirected to Log In or Create an Account during the process of creating your quote.
STEP 2: Add all the products you want quoted into the shopping cart by clicking "ADD TO CART" from each product page.
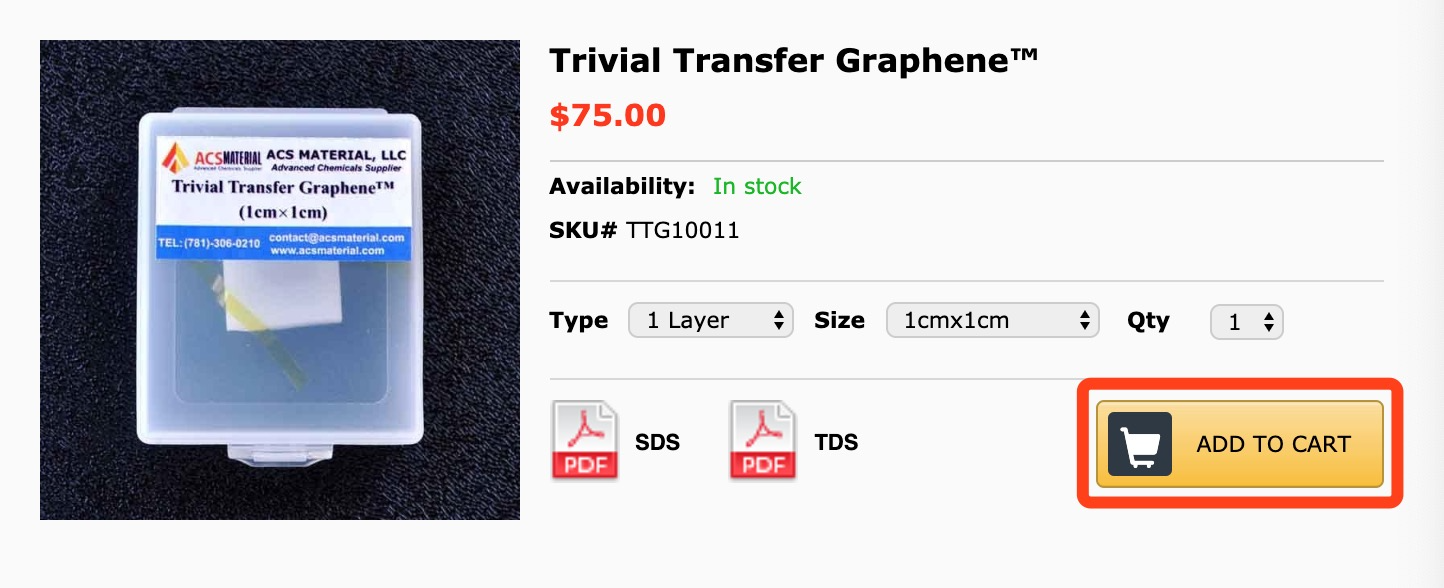
STEP 3: Click the shopping cart icon and review the items in your cart. Click "GET A QUOTE" when you are ready to proceed.
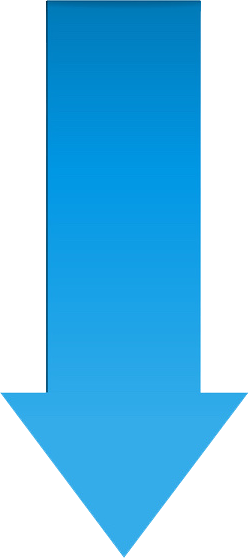
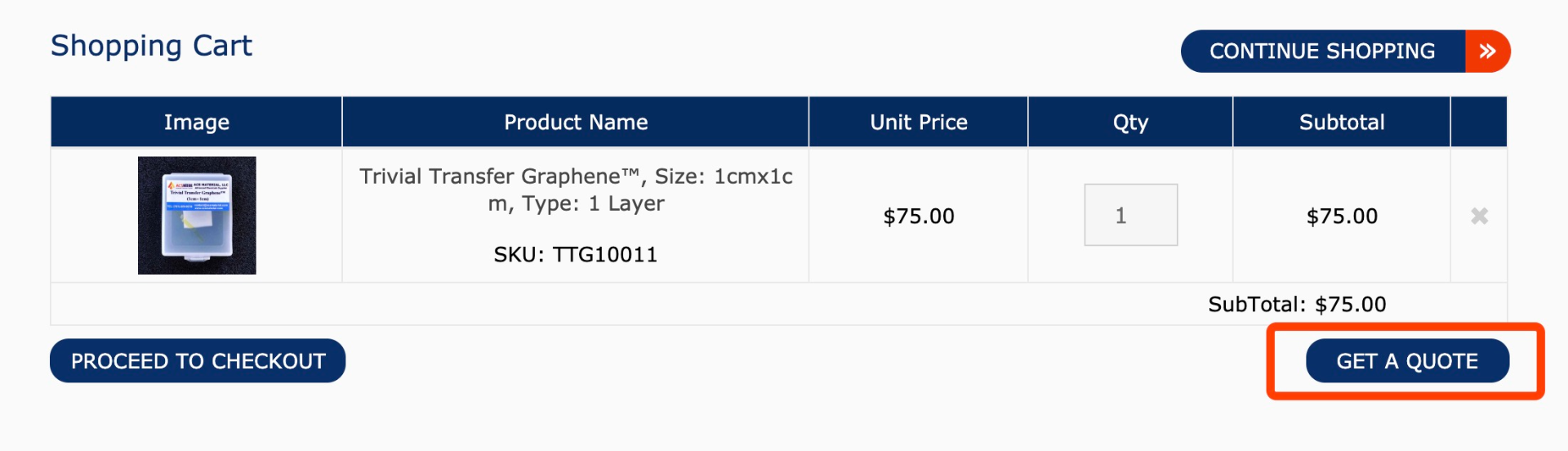
If you are not already logged in, you will be redirected to Log In or Create an Account before proceeding to Step 4.
STEP 4: Fill in all of the required fields and click “GET A QUOTE” at the bottom again.
A. Fill in the required information and choose your shipping speed.
B. If you are planning to pay by credit card, NO NEED to provide your credit card information at this time for creating a quote. You may leave this part blank! Be sure to click the box if you are tax exempt.
C. Click "GET A QUOTE".
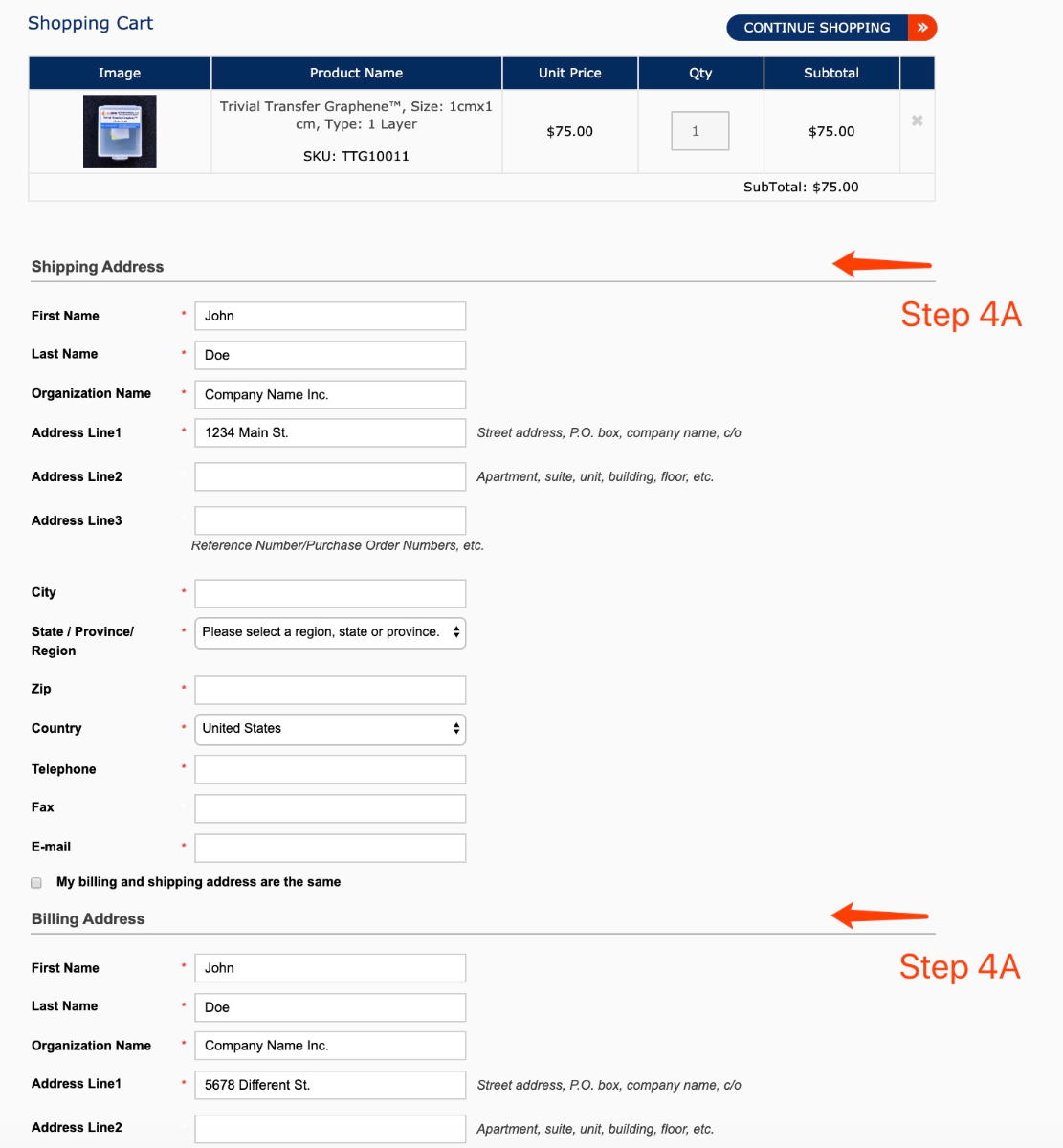
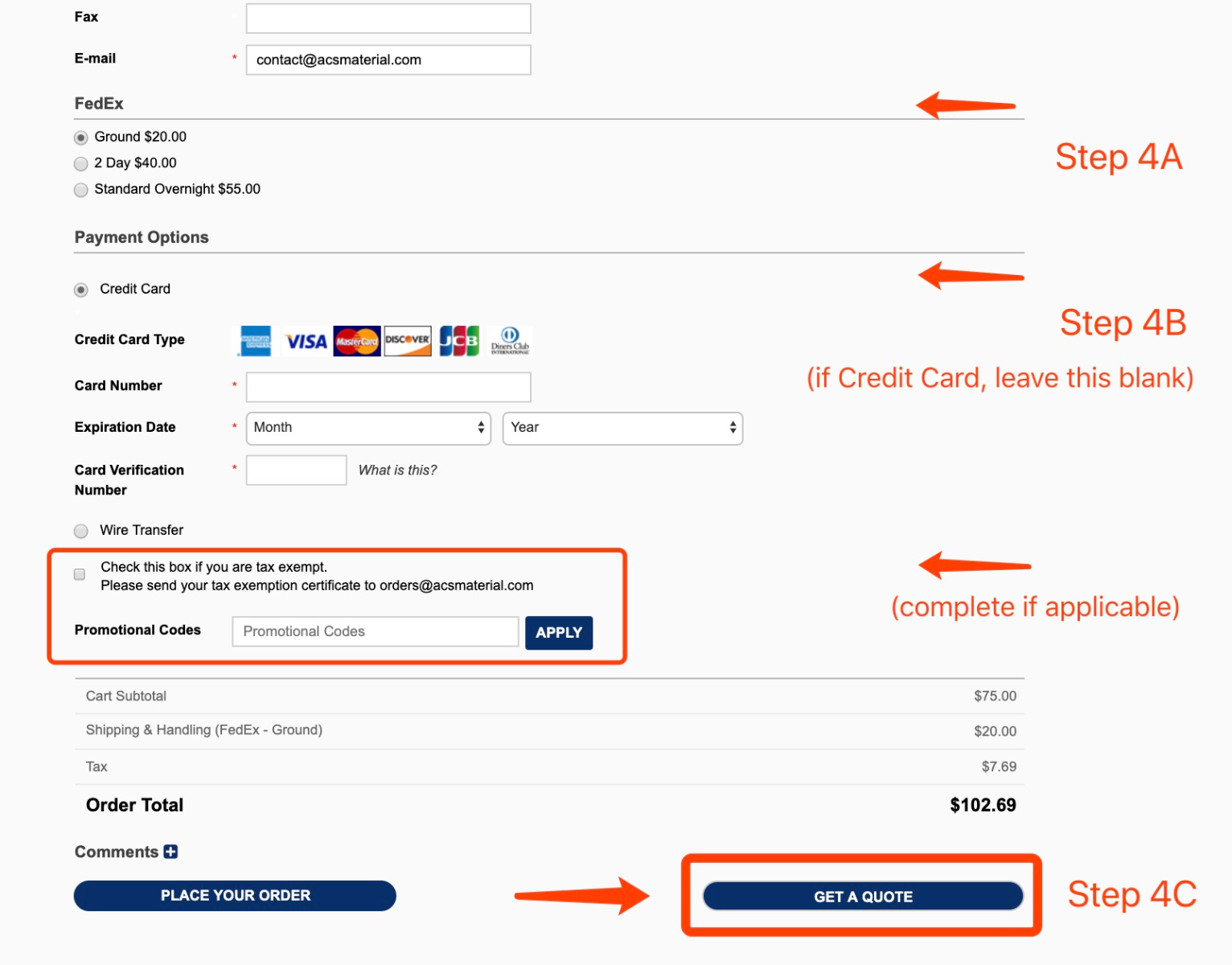
|
-
STEP 1: Have an account with ACS already? Please log in first.
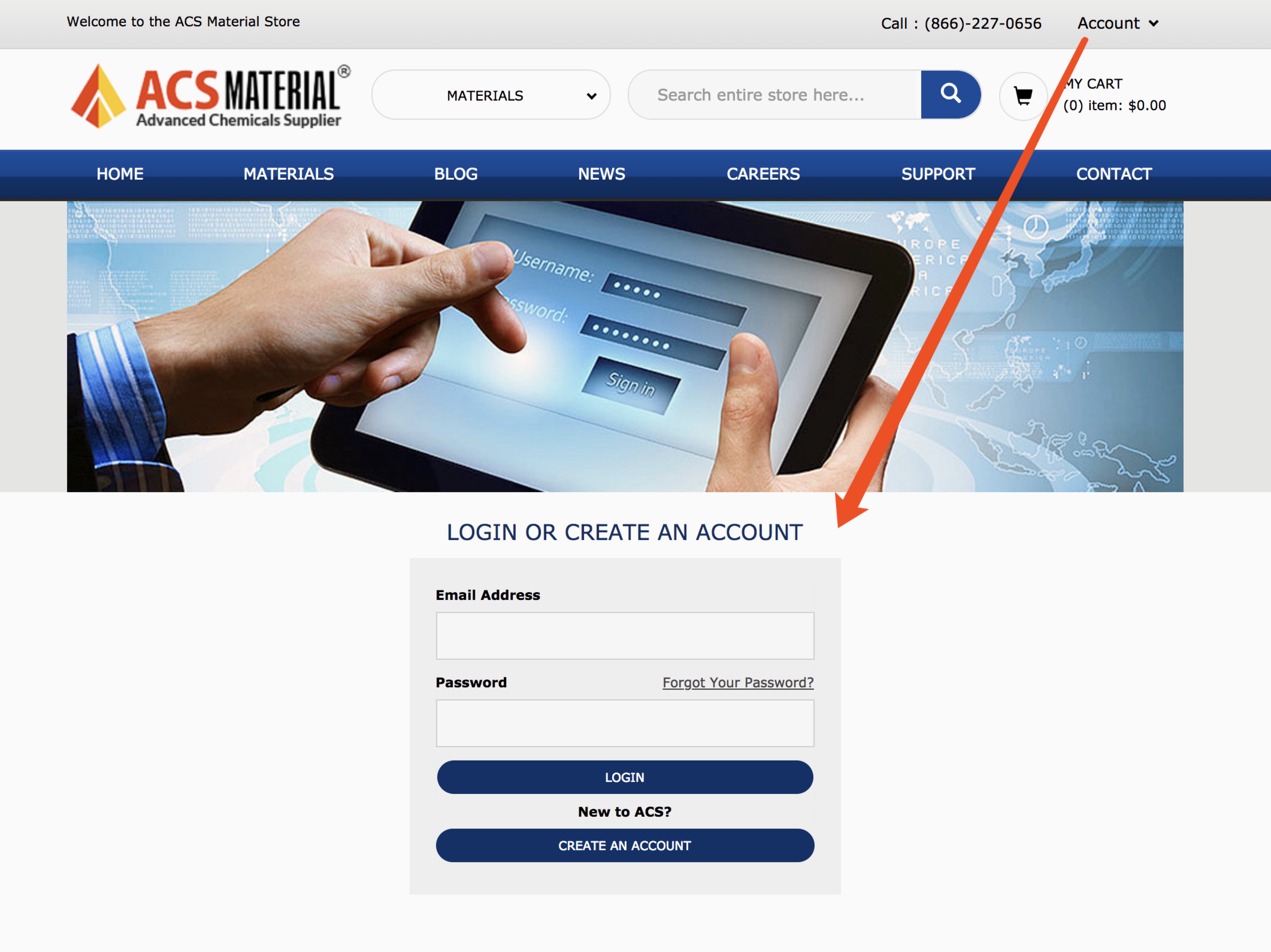
New to ACS Material? Please create an account first.
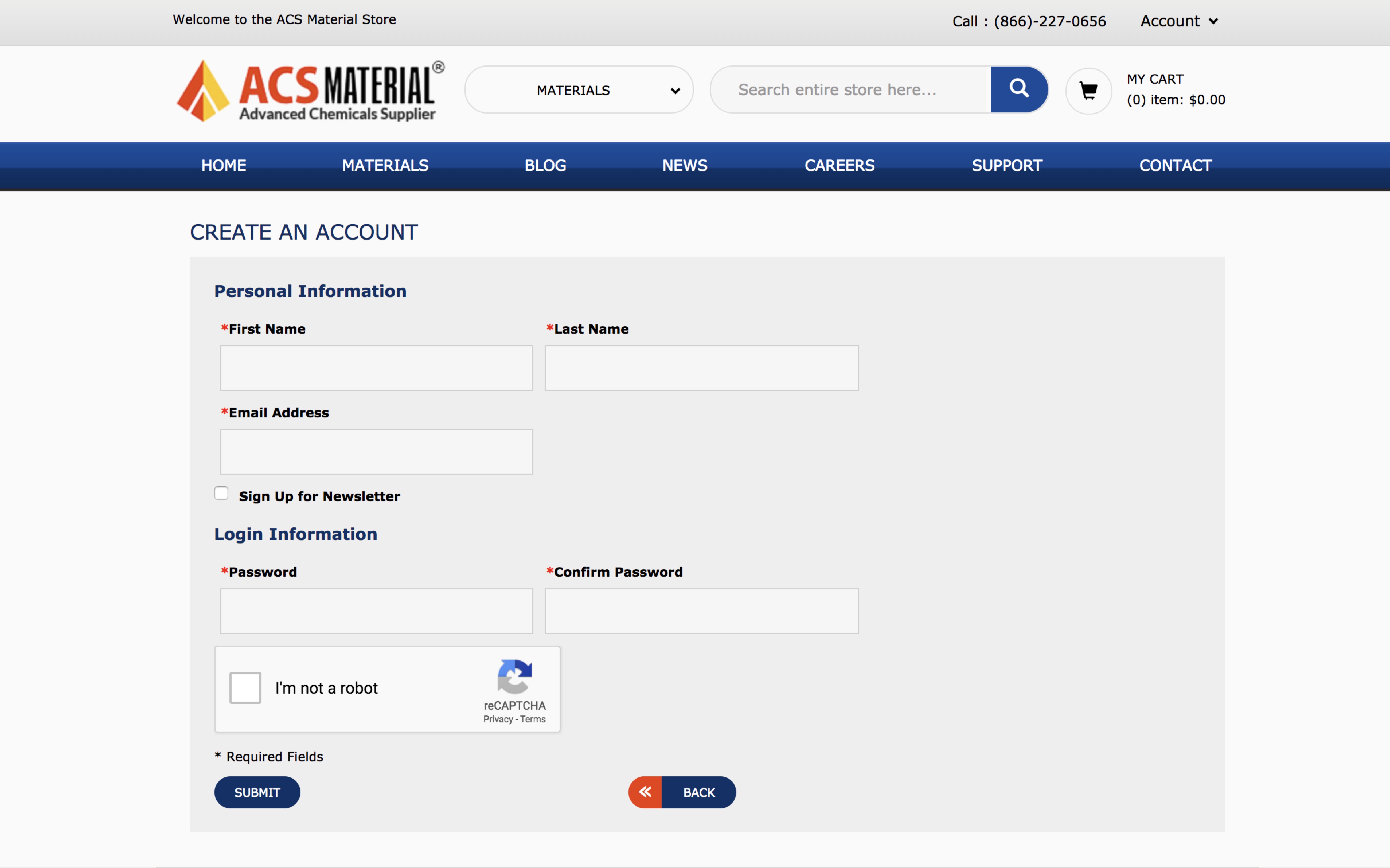
STEP 2: Browse through our Materials Catalog or use keywords in the search bar to find the products you need.
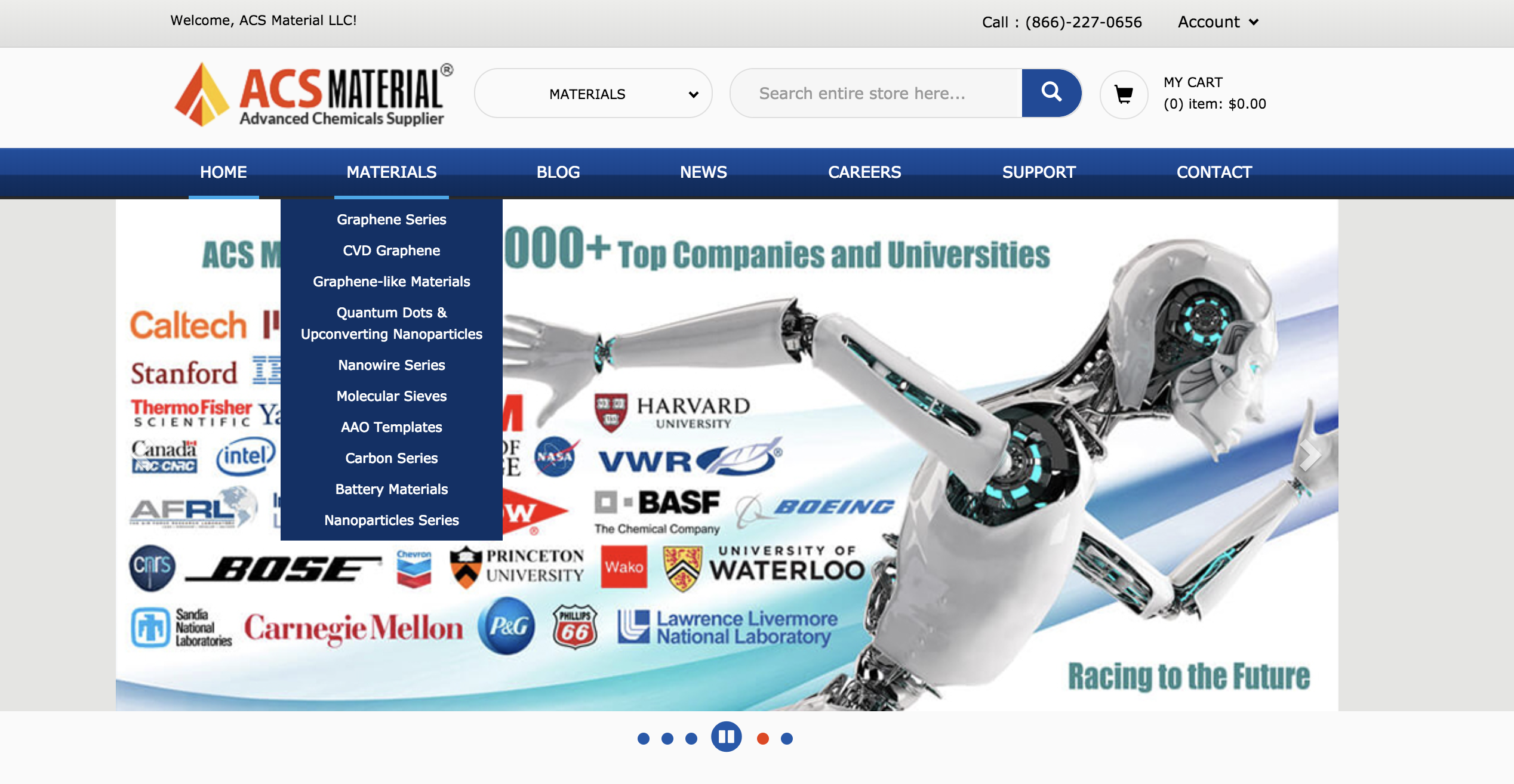
STEP 3: Specify the type, package size and quantity you want to purchase then ADD TO CART.
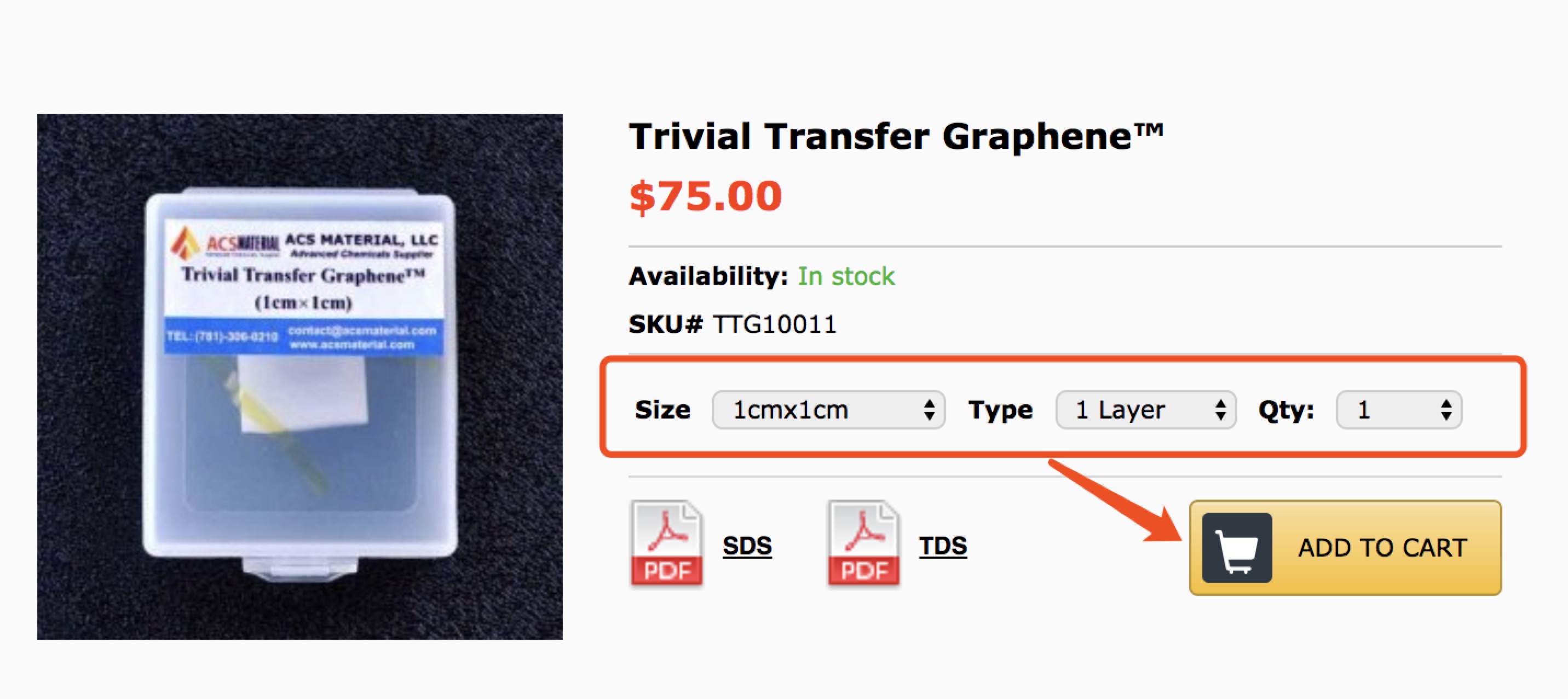
STEP 4: Review the items in your Shopping Cart by clicking on the Shopping Cart icon at the top of your screen.
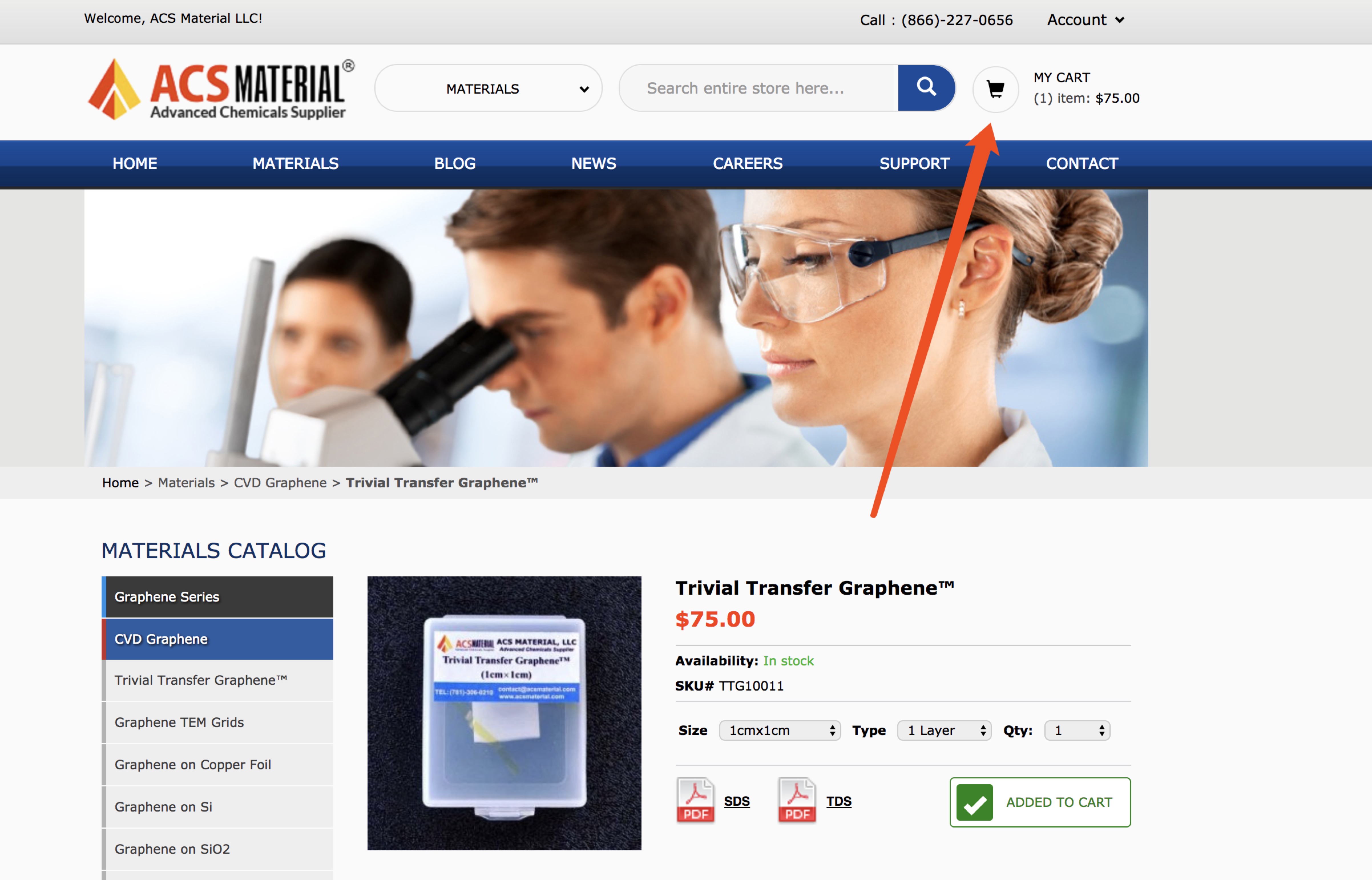
STEP 5: Fill out your billing and shipping addresses(Part A). Choose your preferred shipping method(Part B) and payment option(Part C).
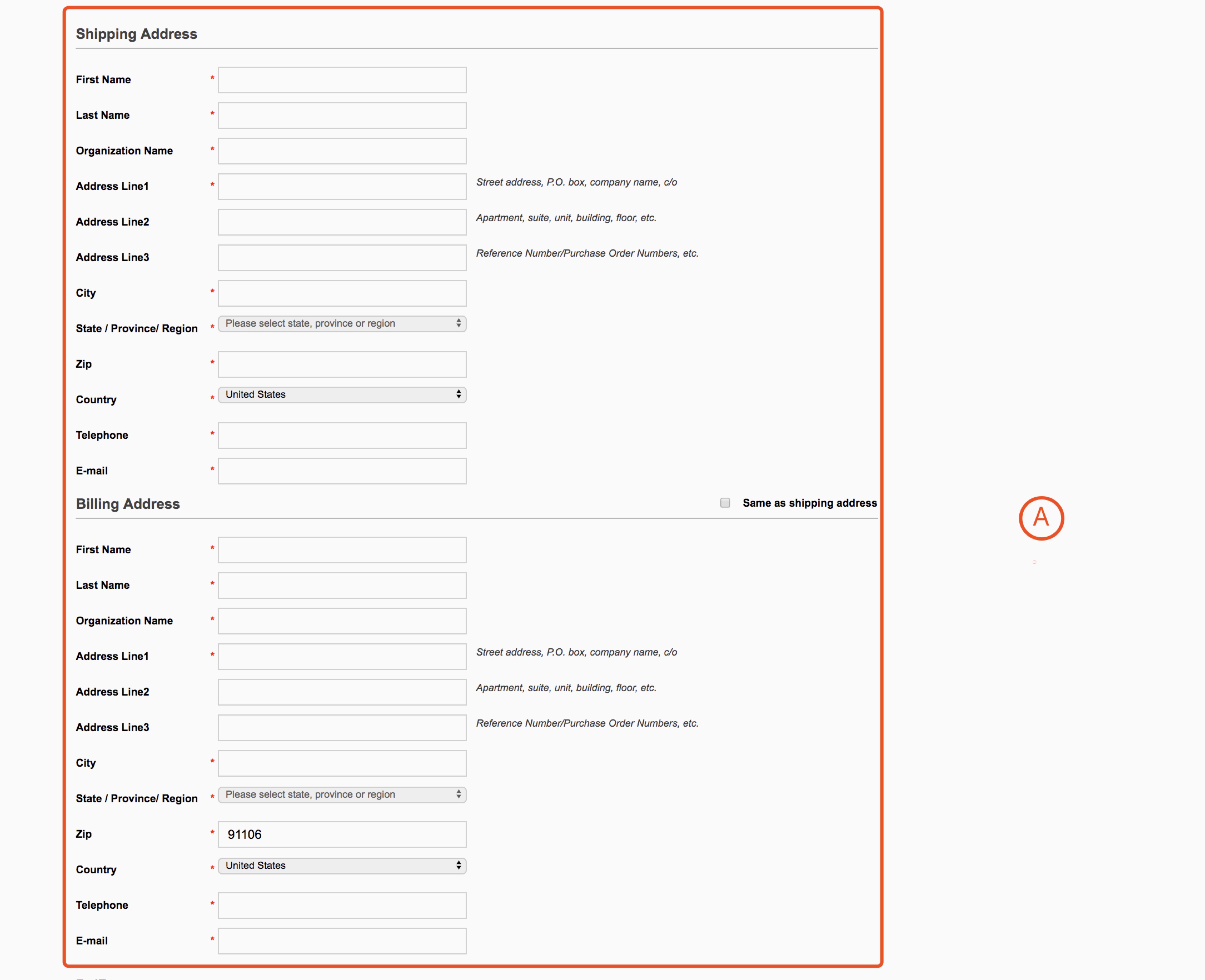
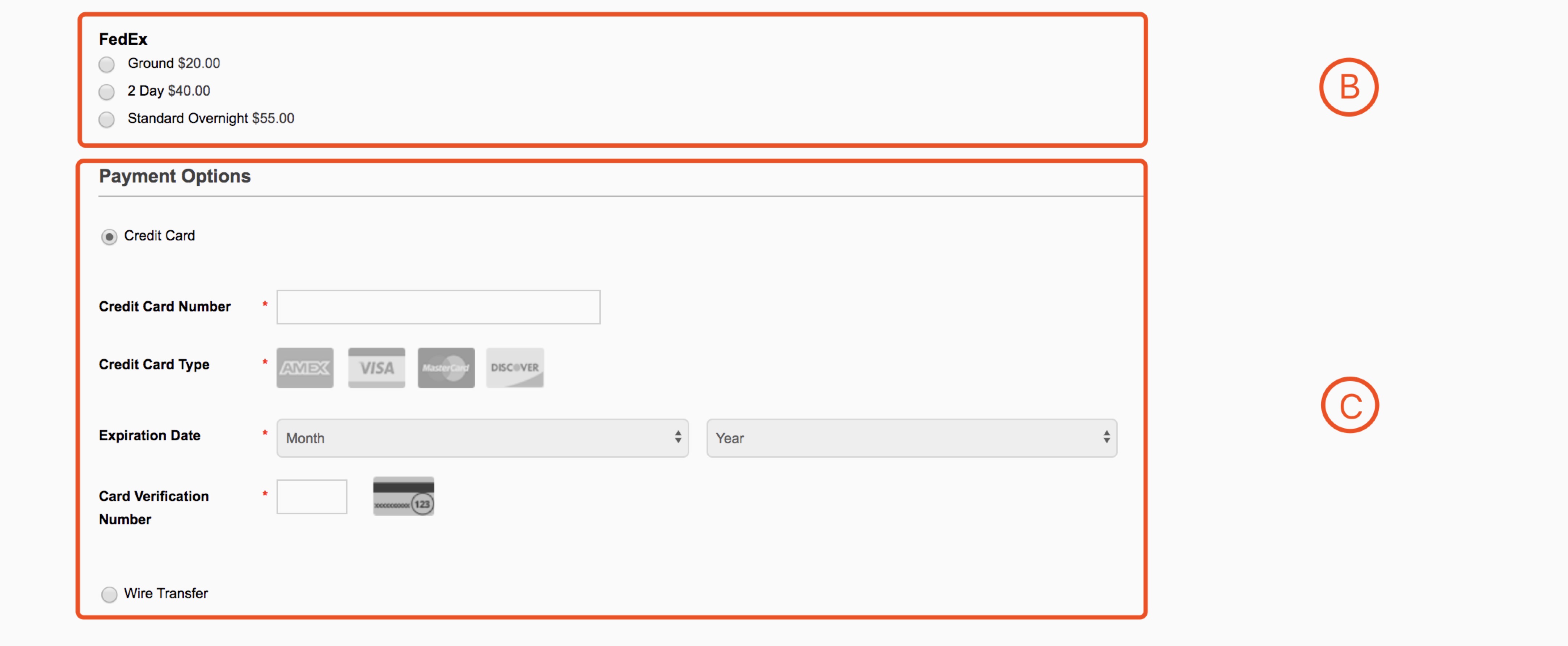
STEP 6: Click PLACE YOUR ORDER. Alternatively, you can use the same form to create an automated quote if you click GET A QUOTE instead. You can also add Comments if needed.
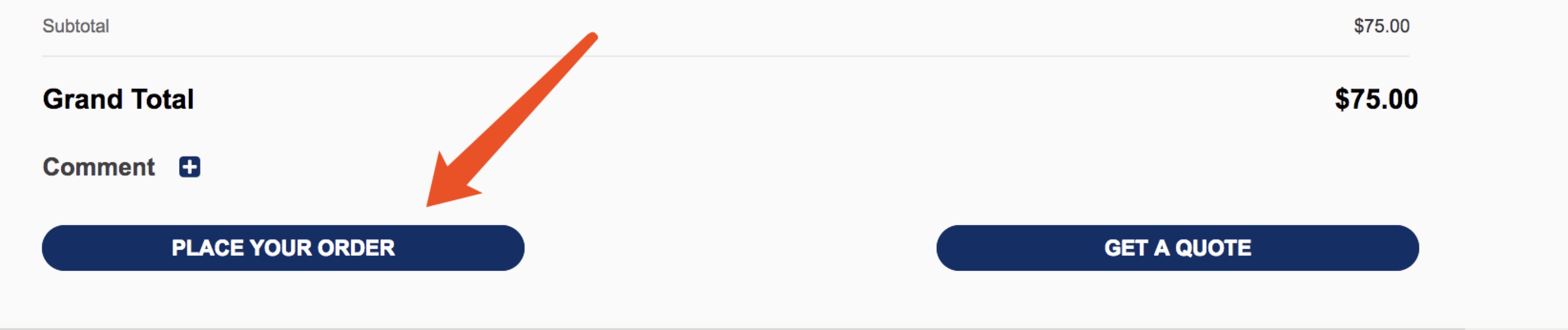
STEP 7: You will receive an order confirmation email shortly thereafter and another shipping notification email in 1-2 days with the tracking number.
|
-
FAQ
1. What is the average pore size?
The average pore size is about ~0.2mm
2. What are the number of layers of graphene?
The graphene layers are from a few layers to dozens of layers. Generally if the layers are too thin, then it will collapse.
3. Has this foam used for the synthesis of metal-graphene composites?
Yes, this foam has been used and can do metal-graphene composite preparation, but kindly note that many specific experiments may need their own assessment depending on the application.
4. What is the thickness of Ni wire substrate used?
The thickness of the nickel foam substrate is 1mm thick.
Research Citations of ACS Material Products
- Lin, Huan, et al. “Significantly reduced thermal diffusivity of free-Standing two-Layer graphene in graphene foam.” Nanotechnology, vol. 24, no. 41, 2013, p. 415706., doi:10.1088/0957-4484/24/41/415706.
- Asfaw, Habtom D., et al. “Nanosized LiFePO4-Decorated emulsion-Templated carbon foam for 3D micro batteries: a study of structure and electrochemical performance.” Nanoscale, vol. 6, no. 15, 2014, pp. 8804–8813., doi:10.1039/c4nr01682c.
- Leeuwner, M. J., et al. “Novel Graphene Foam Microporous Layers for PEM Fuel Cells: Interfacial Characteristics and Comparative Performance.” Fuel Cells, vol. 15, no. 6, Mar. 2015, pp. 790–801., doi:10.1002/fuce.201500031.
- Nieto, Andy, et al. “Graphene reinforced metal and ceramic matrix composites: a review.” International Materials Reviews, vol. 62, no. 5, 27 Oct. 2016, doi:10.1080/09506608.2016.1219481.
|
-
FAQ
1. What is the average pore size?
The average pore size is about ~0.2mm
2. What are the number of layers of graphene?
The graphene layers are from a few layers to dozens of layers. Generally if the layers are too thin, then it will collapse.
3. Has this foam used for the synthesis of metal-graphene composites?
Yes, this foam has been used and can do metal-graphene composite preparation, but kindly note that many specific experiments may need their own assessment depending on the application.
Research Citations of ACS Material Products
- Lin, Huan, et al. “Significantly reduced thermal diffusivity of free-Standing two-Layer graphene in graphene foam.” Nanotechnology, vol. 24, no. 41, 2013, p. 415706., doi:10.1088/0957-4484/24/41/415706.
- Asfaw, Habtom D., et al. “Nanosized LiFePO4-Decorated emulsion-Templated carbon foam for 3D micro batteries: a study of structure and electrochemical performance.” Nanoscale, vol. 6, no. 15, 2014, pp. 8804–8813., doi:10.1039/c4nr01682c.
- Leeuwner, M. J., et al. “Novel Graphene Foam Microporous Layers for PEM Fuel Cells: Interfacial Characteristics and Comparative Performance.” Fuel Cells, vol. 15, no. 6, Mar. 2015, pp. 790–801., doi:10.1002/fuce.201500031.
- Nieto, Andy, et al. “Graphene reinforced metal and ceramic matrix composites: a review.” International Materials Reviews, vol. 62, no. 5, 27 Oct. 2016, doi:10.1080/09506608.2016.1219481.
|
-
Research Citations of ACS Material Products
- Drobná, Helena, et al. “Analysis of Ni species formed on zeolites, mesoporous silica and alumina supports and their catalytic behavior in the dry reforming of methane.” Reaction Kinetics, Mechanisms and Catalysis, vol. 121, no. 1, Sept. 2017, pp. 255–274., doi:10.1007/s11144-017-1149-3.
- Jibril, Zainab Ibrahim, Anita Ramli, and Khairulazhar Jumbri. "Al-MCM-41 Based Catalysts for Transesterification of Jatropha Oil to Biodiesel: Effect of Ce and Zr." Journal of the Japan Institute of Energy 97, no. 7 (2018): 200-204.
- Jibril, Z. I., A. Ramli, and K. Jumbri. "Preparation and Characterization of Ce-Zr/Al-MCM-41 Bimetallic Catalyst for Transesterification of Jatropha Oil to Biodiesel." In IOP Conference Series: Earth and Environmental Science, vol. 168, no. 1, p. 012013. IOP Publishing, 2018.
|
-
FAQ
1. Is this product made from graphene or graphene oxide?
The aminated graphene octadecylamine covalently linked is made of graphene oxide.
Research Citations of ACS Material Products
- Nieto, Andy, et al. “Graphene reinforced metal and ceramic matrix composites: a review.” International Materials Reviews, vol. 62, no. 5, 2016, pp. 241–302., doi:10.1080/09506608.2016.1219481.
- Gago, Israel, et al. “Graphene-Based nanocomposite for body armour: tensile, impact and bulletproof properties.” Nov. 2017.
|
-
Research Citations of ACS Material Products
- Nieto, Andy, et al. “Graphene reinforced metal and ceramic matrix composites: a review.” International Materials Reviews, vol. 62, no. 5, 2016, pp. 241–302., doi:10.1080/09506608.2016.1219481.
|
-
FAQ
1. Are the Aminated Graphene Quantum Dots positively or negatively charged on the surface?
Aminated Graphene Quantum Dots have negative charges. Aminated Graphene Quantum Dots are modified on the surface to provide positive charge. However, the surface contains not only amino groups, but also carboxyl and hydroxyl groups. These negative charged groups do not fully participate in the reaction, so the surface indicates a negative charge.
Research Citations of ACS Material Products
- Li, Changzheng, and Yanan Yue. “Fluorescence spectroscopy of graphene quantum dots: temperature effect at different excitation wavelengths.” Nanotechnology, vol. 25, no. 43, Sept. 2014, p. 435703., doi:10.1088/0957-4484/25/43/435703.
- Bhatnagar, Deepika, et al. “Graphene quantum dots FRET based sensor for early detection of heart attack in human.” Biosensors and Bioelectronics, vol. 79, 2016, pp. 495–499., doi:10.1016/j.bios.2015.12.083.
- Liu, Yang, et al. “Electro-Optical switching of liquid crystals sandwiched between ion-Beam-Spurted graphene quantum dots-Doped PEDOT:PSS composite layers.” Optics Express, vol. 23, no. 26, 2015, p. 34071., doi:10.1364/oe.23.034071.
- Bhatnagar, Deepika, et al. “Ultrasensitive cardiac troponin I antibody based nanohybrid sensor for rapid detection of human heart attack.” International Journal of Biological Macromolecules, vol. 95, 2017, pp. 505–510., doi:10.1016/j.ijbiomac.2016.11.037.
- Nieto, Andy, et al. “Graphene reinforced metal and ceramic matrix composites: a review.” International Materials Reviews, vol. 62, no. 5, 2016, pp. 241–302., doi:10.1080/09506608.2016.1219481.
- Bok, Chang Han, et al. “Operating mechanisms of highly-Reproducible write-Once-Read-Many-Times memory devices based on graphene quantum dot:Poly(Methyl silsesquioxane) nanocomposites.” Applied Physics Letters, vol. 110, no. 1, Apr. 2017, p. 013301., doi:10.1063/1.4973358.
- Kim, Do Hyeong, et al. “Highly-Reproducible nonvolatile memristive devices based on polyvinylpyrrolidone: Graphene quantum-Dot nanocomposites.” Organic Electronics, vol. 51, 2017, pp. 156–161., doi:10.1016/j.orgel.2017.09.005.
- Gupta, Shagun, et al. “Ultrasensitive transglutaminase based nanosensor for early detection of celiac disease in human.” International Journal of Biological Macromolecules, vol. 105, 2017, pp. 905–911., doi:10.1016/j.ijbiomac.2017.07.126.
- Ooi, Poh Choon, et al. “Reduced graphene oxide preparation and its applications in solution-Processed write-Once-Read-Many-Times graphene-Based memory device.” Carbon, vol. 124, 2017, pp. 547–554., doi:10.1016/j.carbon.2017.09.004.
- Choi, Hwan Young, et al. “Organic electronic synapses with pinched hystereses based on graphene quantum-Dot nanocomposites.” NPG Asia Materials, vol. 9, no. 7, 2017, doi:10.1038/am.2017.133.
- Ooi, Poh Choon, et al. “Fabrication of transparent bistable switching memory device using plasmapolymerized hexamethyldisiloxane layers with embedded graphene quantum dots.” Thin Solid Films, vol. 645, 2018, pp. 45–50., doi:10.1016/j.tsf.2017.10.044.
- Wang, Changhong, et al. “Computing: Memristive Devices with Highly Repeatable Analog States Boosted by Graphene Quantum Dots.” Small, vol. 13, no. 20, 2017, doi:10.1002/smll.201770110.
- Bakar, Elyani Abu, Mohd Ambri Mohamed, Poh Choon Ooi, MF Mohd Razip Wee, Chang Fu Dee, and Burhanuddin Yeop Majlis. "Fabrication of indium-tin-oxide free, all-solution-processed flexible nanogenerator device using nanocomposite of barium titanate and graphene quantum dots in polyvinylidene fluoride polymer matrix." Organic Electronics 61 (2018): 289-295.
- Ooi, Poh Choon, MF Mohd Razip Wee, Chang Fu Dee, Chi Chin Yap, Muhammad Mat Salleh, and Burhanuddin Yeop Majlis. "Fabrication of transparent bistable switching memory device using plasmapolymerized hexamethyldisiloxane layers with embedded graphene quantum dots." Thin Solid Films 645 (2018): 45-50.
- Sung, Sihyun, Chaoxing Wu, Hyun Soo Jung, and Tae Whan Kim. "Highly-stable write-once-read-many-times switching behaviors of 1D–1R memristive devices based on graphene quantum dot nanocomposites." Scientific reports 8, no. 1 (2018): 12081.
|
-
Research Citations of ACS Material Products
- Li, Changzheng, and Yanan Yue. “Fluorescence spectroscopy of graphene quantum dots: temperature effect at different excitation wavelengths.” Nanotechnology, vol. 25, no. 43, Sept. 2014, p. 435703., doi:10.1088/0957-4484/25/43/435703.
- Bhatnagar, Deepika, et al. “Graphene quantum dots FRET based sensor for early detection of heart attack in human.” Biosensors and Bioelectronics, vol. 79, 2016, pp. 495–499., doi:10.1016/j.bios.2015.12.083.
- Liu, Yang, et al. “Electro-Optical switching of liquid crystals sandwiched between ion-Beam-Spurted graphene quantum dots-Doped PEDOT:PSS composite layers.” Optics Express, vol. 23, no. 26, 2015, p. 34071., doi:10.1364/oe.23.034071.
- Bhatnagar, Deepika, et al. “Ultrasensitive cardiac troponin I antibody based nanohybrid sensor for rapid detection of human heart attack.” International Journal of Biological Macromolecules, vol. 95, 2017, pp. 505–510., doi:10.1016/j.ijbiomac.2016.11.037.
- Nieto, Andy, et al. “Graphene reinforced metal and ceramic matrix composites: a review.” International Materials Reviews, vol. 62, no. 5, 2016, pp. 241–302., doi:10.1080/09506608.2016.1219481.
- Bok, Chang Han, et al. “Operating mechanisms of highly-Reproducible write-Once-Read-Many-Times memory devices based on graphene quantum dot:Poly(Methyl silsesquioxane) nanocomposites.” Applied Physics Letters, vol. 110, no. 1, Apr. 2017, p. 013301., doi:10.1063/1.4973358.
- Kim, Do Hyeong, et al. “Highly-Reproducible nonvolatile memristive devices based on polyvinylpyrrolidone: Graphene quantum-Dot nanocomposites.” Organic Electronics, vol. 51, 2017, pp. 156–161., doi:10.1016/j.orgel.2017.09.005.
- Gupta, Shagun, et al. “Ultrasensitive transglutaminase based nanosensor for early detection of celiac disease in human.” International Journal of Biological Macromolecules, vol. 105, 2017, pp. 905–911., doi:10.1016/j.ijbiomac.2017.07.126.
- Ooi, Poh Choon, et al. “Reduced graphene oxide preparation and its applications in solution-Processed write-Once-Read-Many-Times graphene-Based memory device.” Carbon, vol. 124, 2017, pp. 547–554., doi:10.1016/j.carbon.2017.09.004.
- Choi, Hwan Young, et al. “Organic electronic synapses with pinched hystereses based on graphene quantum-Dot nanocomposites.” NPG Asia Materials, vol. 9, no. 7, 2017, doi:10.1038/am.2017.133.
- Ooi, Poh Choon, et al. “Fabrication of transparent bistable switching memory device using plasmapolymerized hexamethyldisiloxane layers with embedded graphene quantum dots.” Thin Solid Films, vol. 645, 2018, pp. 45–50., doi:10.1016/j.tsf.2017.10.044.
- Wang, Changhong, et al. “Computing: Memristive Devices with Highly Repeatable Analog States Boosted by Graphene Quantum Dots.” Small, vol. 13, no. 20, 2017, doi:10.1002/smll.201770110.
- Bakar, Elyani Abu, Mohd Ambri Mohamed, Poh Choon Ooi, MF Mohd Razip Wee, Chang Fu Dee, and Burhanuddin Yeop Majlis. "Fabrication of indium-tin-oxide free, all-solution-processed flexible nanogenerator device using nanocomposite of barium titanate and graphene quantum dots in polyvinylidene fluoride polymer matrix." Organic Electronics 61 (2018): 289-295.
- Ooi, Poh Choon, MF Mohd Razip Wee, Chang Fu Dee, Chi Chin Yap, Muhammad Mat Salleh, and Burhanuddin Yeop Majlis. "Fabrication of transparent bistable switching memory device using plasmapolymerized hexamethyldisiloxane layers with embedded graphene quantum dots." Thin Solid Films 645 (2018): 45-50.
- Sung, Sihyun, Chaoxing Wu, Hyun Soo Jung, and Tae Whan Kim. "Highly-stable write-once-read-many-times switching behaviors of 1D–1R memristive devices based on graphene quantum dot nanocomposites." Scientific reports 8, no. 1 (2018): 12081.
|
-
FAQ
1. What is the particle size of the Beta Zeolite?
Our Beta Zeolite product has a particle size of 20-30nm.
Research Citations of ACS Material Products
- Rusli, Muhammad Syhamiel Iqhwan Che, et al. “Characterization Of Pcl/Zeolite Electrospun Membrane For The Removal Of Silver In Drinking Water.” Jurnal Teknologi, vol. 79, no. 1-2, 2017, doi:10.11113/jt.v79.10442.
- Sultana, N., et al. “Zeolite Incorporated Polycaprolactone/Zeolite Nanocomposite Membranes for Silver Removal.” Applied Membrane Science & Technology, www.amst.utm.my/index.php/amst/article/view/106.
- Güleç, Fatih, Farooq Sher, and Ali Karaduman. "Catalytic performance of Cu-and Zr-modified beta zeolite catalysts in the methylation of 2-methylnaphthalene." Petroleum Science 16, no. 1 (2019): 161-172.
- Brown, Erika P., Sijia Hu, Jon Wells, Xiaohui Wang, and Carolyn A. Koh. "Direct measurements of contact angles on cyclopentane hydrates." Energy & fuels 32, no. 6 (2018): 6619-6626.
|
-
Research Citations of ACS Material Products
- Wang, Sheng, et al. “Black Phosphorus Nanosheets for Mild Hyperthermia-Enhanced Chemotherapy and Chemo-Photothermal Combination Therapy.” Nanotheranostics, vol. 1, no. 2, 2017, pp. 208–216., doi:10.7150/ntno.18767.
- Park, Jin Woo, et al. “Layer-Controlled thinning of black phosphorus by an Ar ion beam.” J. Mater. Chem. C, vol. 5, no. 41, 2017, pp. 10888–10893., doi:10.1039/c7tc03101g.
- Lazar, Petr, Eva Otyepková, Martin Pykal, Klára Čépe, and Michal Otyepka. "Role of the puckered anisotropic surface in the surface and adsorption properties of black phosphorus." Nanoscale 10, no. 19 (2018): 8979-8988.
|
-
Research Citations of ACS Material Products
- Li, Changzheng, and Yanan Yue. “Fluorescence spectroscopy of graphene quantum dots: temperature effect at different excitation wavelengths.” Nanotechnology, vol. 25, no. 43, Sept. 2014, p. 435703., doi:10.1088/0957-4484/25/43/435703.
- Bhatnagar, Deepika, et al. “Graphene quantum dots FRET based sensor for early detection of heart attack in human.” Biosensors and Bioelectronics, vol. 79, 2016, pp. 495–499., doi:10.1016/j.bios.2015.12.083.
- Liu, Yang, et al. “Electro-Optical switching of liquid crystals sandwiched between ion-Beam-Spurted graphene quantum dots-Doped PEDOT:PSS composite layers.” Optics Express, vol. 23, no. 26, 2015, p. 34071., doi:10.1364/oe.23.034071.
- Bhatnagar, Deepika, et al. “Ultrasensitive cardiac troponin I antibody based nanohybrid sensor for rapid detection of human heart attack.” International Journal of Biological Macromolecules, vol. 95, 2017, pp. 505–510., doi:10.1016/j.ijbiomac.2016.11.037.
- Nieto, Andy, et al. “Graphene reinforced metal and ceramic matrix composites: a review.” International Materials Reviews, vol. 62, no. 5, 2016, pp. 241–302., doi:10.1080/09506608.2016.1219481.
- Bok, Chang Han, et al. “Operating mechanisms of highly-Reproducible write-Once-Read-Many-Times memory devices based on graphene quantum dot:Poly(Methyl silsesquioxane) nanocomposites.” Applied Physics Letters, vol. 110, no. 1, Apr. 2017, p. 013301., doi:10.1063/1.4973358.
- Kim, Do Hyeong, et al. “Highly-Reproducible nonvolatile memristive devices based on polyvinylpyrrolidone: Graphene quantum-Dot nanocomposites.” Organic Electronics, vol. 51, 2017, pp. 156–161., doi:10.1016/j.orgel.2017.09.005.
- Gupta, Shagun, et al. “Ultrasensitive transglutaminase based nanosensor for early detection of celiac disease in human.” International Journal of Biological Macromolecules, vol. 105, 2017, pp. 905–911., doi:10.1016/j.ijbiomac.2017.07.126.
- Ooi, Poh Choon, et al. “Reduced graphene oxide preparation and its applications in solution-Processed write-Once-Read-Many-Times graphene-Based memory device.” Carbon, vol. 124, 2017, pp. 547–554., doi:10.1016/j.carbon.2017.09.004.
- Choi, Hwan Young, et al. “Organic electronic synapses with pinched hystereses based on graphene quantum-Dot nanocomposites.” NPG Asia Materials, vol. 9, no. 7, 2017, doi:10.1038/am.2017.133.
- Ooi, Poh Choon, et al. “Fabrication of transparent bistable switching memory device using plasmapolymerized hexamethyldisiloxane layers with embedded graphene quantum dots.” Thin Solid Films, vol. 645, 2018, pp. 45–50., doi:10.1016/j.tsf.2017.10.044.
- Wang, Changhong, et al. “Computing: Memristive Devices with Highly Repeatable Analog States Boosted by Graphene Quantum Dots.” Small, vol. 13, no. 20, 2017, doi:10.1002/smll.201770110.
- Bakar, Elyani Abu, Mohd Ambri Mohamed, Poh Choon Ooi, MF Mohd Razip Wee, Chang Fu Dee, and Burhanuddin Yeop Majlis. "Fabrication of indium-tin-oxide free, all-solution-processed flexible nanogenerator device using nanocomposite of barium titanate and graphene quantum dots in polyvinylidene fluoride polymer matrix." Organic Electronics 61 (2018): 289-295.
- Ooi, Poh Choon, MF Mohd Razip Wee, Chang Fu Dee, Chi Chin Yap, Muhammad Mat Salleh, and Burhanuddin Yeop Majlis. "Fabrication of transparent bistable switching memory device using plasmapolymerized hexamethyldisiloxane layers with embedded graphene quantum dots." Thin Solid Films 645 (2018): 45-50.
- Sung, Sihyun, Chaoxing Wu, Hyun Soo Jung, and Tae Whan Kim. "Highly-stable write-once-read-many-times switching behaviors of 1D–1R memristive devices based on graphene quantum dot nanocomposites." Scientific reports 8, no. 1 (2018): 12081.
|
-
FAQ
1. What is the effect on properties of increasing NH2 content? In other words, is 0.45 wt.% standardized?
If the content of amino groups is increased, this system will have compatibility with amino groups, and the compatibility of the entire system will be higher than before.
2. Will that NH2 content be good enough for stable dispersion in polar solvent?
We suspect this would be not only a matter of the amino group, but the two most important aspects are the dispersant and the dispersing equipment.
|
|
-
How you get the graphene in the first place? Is it through Hummer's method‚ hydrazine treatment and then some method of functionalization or maybe a different route?
ACS Material prepares Graphene Oxide using the Modified Hummer’s Method. The synthesis following that is proprietary and we cannot disclose the details‚ but we are able to produce the functional group: -COOH as a stock item‚ and the groups: -NH‚ -NH2‚ -Amino-PEG‚ and rGO-NH-Carboimidazole as special order items. Typically‚ the (-COOH) can be produced by organic reaction using (-OH) and (C-O-C).
What is the structure of Carboxyl Graphene? The carboxyl groups are only at edges‚ or they are also attached to the basil plane of graphene? What is the electrical conductivity of this product?
Most Carboxyl groups (-COOH) are at the edges. The other related group present is derived from –OH or C-O-H which yields O-CH2-COOH. Carboxyl Graphene is non-conductive.
Research Citations of ACS Material Products
- Lammel, Tobias, et al. “Internalization and cytotoxicity of graphene oxide and carboxyl graphene nanoplatelets in the human hepatocellular carcinoma cell line Hep G2.” Particle and Fibre Toxicology, vol. 10, no. 1, 2013, p. 27., doi:10.1186/1743-8977-10-27.
- Chu, Bryan, et al. “Graphene-Enhanced Environmentally-Benign Cutting Fluids for High-Performance Micro-Machining Applications.” Journal of Nanoscience and Nanotechnology, vol. 13, no. 8, Jan. 2013, pp. 5500–5504., doi:10.1166/jnn.2013.7538.
- Lammel, Tobias, and José M. Navas. “Graphene nanoplatelets spontaneously translocate into the cytosol and physically interact with cellular organelles in the fish cell line PLHC-1.” Aquatic Toxicology, vol. 150, 2014, pp. 55–65., doi:10.1016/j.aquatox.2014.02.016.
- Lammel, Tobias, et al. “Potentiating effect of graphene nanomaterials on aromatic environmental pollutant-Induced cytochrome P450 1A expression in the topminnow fish hepatoma cell line PLHC-1.” Environmental Toxicology, vol. 30, no. 10, May 2014, pp. 1192–1204., doi:10.1002/tox.21991.
- Liu, Zhenbao, et al. “Intracellular Detection of ATP Using an Aptamer Beacon Covalently Linked to Graphene Oxide Resisting Nonspecific Probe Displacement.” Analytical Chemistry, vol. 86, no. 24, 2014, pp. 12229–12235., doi:10.1021/ac503358m.
- Robertson, Neil M., et al. “Monitoring the Multitask Mechanism of DNase I Activity Using Graphene Nanoassemblies.” Bioconjugate Chemistry, vol. 26, no. 4, Oct. 2015, pp. 735–745., doi:10.1021/acs.bioconjchem.5b00067.
- Balcioglu, Mustafa, et al. “Doxorubicin loading on graphene oxide, iron oxide and gold nanoparticle hybrid.” Journal of Materials Chemistry B, vol. 1, no. 45, 2013, p. 6187., doi:10.1039/c3tb20992j.
- Rana, Muhit, et al. “Nano-Graphene oxide as a novel platform for monitoring the effect of LNA modification on nucleic acid interactions.” The Analyst, vol. 139, no. 4, 2014, pp. 714–720., doi:10.1039/c3an02066e.
- Kaplan, Amir, et al. “Structures Self-Assembled from Anionic Graphene and Cationic Manganese Porphyrin: Characterization and Application in Artificial Photosynthesis.” European Journal of Inorganic Chemistry, vol. 2014, no. 13, 2014, pp. 2288–2295., doi:10.1002/ejic.201400054.
- Balcioglu, Mustafa, et al. “DNA-Length-Dependent Quenching of Fluorescently Labeled Iron Oxide Nanoparticles with Gold, Graphene Oxide and MoS2 Nanostructures.” ACS Applied Materials & Interfaces, vol. 6, no. 15, 2014, pp. 12100–12110., doi:10.1021/am503553h.
- Balcioglu, Mustafa, et al. “Smart-Polymer-Functionalized Graphene Nanodevices for Thermo-Switch-Controlled Biodetection.” ACS Biomaterials Science & Engineering, vol. 1, no. 1, Dec. 2015, pp. 27–36., doi:10.1021/ab500029h.
- Lu, Chang, et al. “Covalent linking DNA to graphene oxide and its comparison with physisorbed probes for Hg 2 detection.” Biosensors and Bioelectronics, vol. 79, 2016, pp. 244–250., doi:10.1016/j.bios.2015.12.043.
- Mauro, Nicolò, et al. “Biotin-Containing Reduced Graphene Oxide-Based Nanosystem as a Multieffect Anticancer Agent: Combining Hyperthermia with Targeted Chemotherapy.” Biomacromolecules, vol. 16, no. 9, May 2015, pp. 2766–2775., doi:10.1021/acs.biomac.5b00705.
- Robertson, Neil M., et al. “Discriminating a Single Nucleotide Difference for Enhanced miRNA Detection Using Tunable Graphene and Oligonucleotide Nanodevices.” Langmuir, vol. 31, no. 36, Feb. 2015, pp. 9943–9952., doi:10.1021/acs.langmuir.5b02026.
- Robertson, Neil M., et al. “Unlocked Nucleic Acids for miRNA detection using two dimensional nano-Graphene oxide.” Biosensors and Bioelectronics, vol. 89, 2017, pp. 551–557., doi:10.1016/j.bios.2016.02.058.
- Lu, Chang, et al. “Comparison of Graphene Oxide and Reduced Graphene Oxide for DNA Adsorption and Sensing.” Langmuir, vol. 32, no. 41, June 2016, pp. 10776–10783., doi:10.1021/acs.langmuir.6b03032.
- Ionita, Mariana, et al. “Effect of carboxylic acid functionalized graphene on physical-Chemical and biological performances of polysulfone porous films.” Polymer, vol. 92, 2016, pp. 1–12., doi:10.1016/j.polymer.2016.03.040.
- Nieto, Andy, et al. “Graphene reinforced metal and ceramic matrix composites: a review.” International Materials Reviews, vol. 62, no. 5, 2016, pp. 241–302., doi:10.1080/09506608.2016.1219481.
- Lu, Chang, et al. “Comparison of MoS2, WS2, and Graphene Oxide for DNA Adsorption and Sensing.” Langmuir, vol. 33, no. 2, May 2017, pp. 630–637., doi:10.1021/acs.langmuir.6b04502.
- Qin, Ailin, et al. “Precipitation of PEG/Carboxyl-Modified Gold Nanoparticles with Magnesium Pyrophosphate: A New Platform for Real-Time Monitoring of Loop-Mediated Isothermal Amplification.” ACS Applied Materials & Interfaces, vol. 9, no. 12, 2017, pp. 10472–10480., doi:10.1021/acsami.7b00046.
- Hizir, Mustafa Salih, et al. “Universal sensor array for highly selective system identification using two-Dimensional nanoparticles.” Chemical Science, vol. 8, no. 8, 2017, pp. 5735–5745., doi:10.1039/c7sc01522d.
- Huang, Zhicheng, and Juewen Liu. “Length-Dependent Diblock DNA with Poly-Cytosine (Poly-C) as High-Affinity Anchors on Graphene Oxide.” Langmuir, vol. 34, no. 3, Sept. 2017, pp. 1171–1177., doi:10.1021/acs.langmuir.7b02812.
- Ahmed, Syed Rahin, et al. “Optoelectronic fowl adenovirus detection based on local electric field enhancement on graphene quantum dots and gold nanobundle hybrid.” Biosensors and Bioelectronics, vol. 103, 2018, pp. 45–53., doi:10.1016/j.bios.2017.12.028.
- Wang, Liu, et al. “Transition Metal Dichalcogenide Nanosheets for Visual Monitoring PCR Rivaling a Real-Time PCR Instrument.” ACS Applied Materials & Interfaces, vol. 10, no. 5, 2018, pp. 4409–4418., doi:10.1021/acsami.7b15746.
- Su, Weitao, Naresh Kumar, Andrey Krayev, and Marc Chaigneau. "In situ topographical chemical and electrical imaging of carboxyl graphene oxide at the nanoscale." Nature communications 9, no. 1 (2018): 2891.
- Wang, Liu, Zhicheng Huang, Yibo Liu, Jian Wu, and Juewen Liu. "Fluorescent DNA Probing Nanoscale MnO2: Adsorption, Dissolution by Thiol, and Nanozyme Activity." Langmuir 34, no. 9 (2018): 3094-3101.
- Zhang, Zijie, and Juewen Liu. "An engineered one-site aptamer with higher sensitivity for label-free detection of adenosine on graphene oxide." Canadian Journal of Chemistry 96, no. 11 (2018): 957-963.
- Chand, Rohit, Yi Lan Wang, David Kelton, and Suresh Neethirajan. "Isothermal DNA amplification with functionalized graphene and nanoparticle assisted electroanalysis for rapid detection of Johne’s disease." Sensors and Actuators B: Chemical 261 (2018): 31-37.
|
-
Research Citations of ACS Material Products
- Lammel, Tobias, et al. “Internalization and cytotoxicity of graphene oxide and carboxyl graphene nanoplatelets in the human hepatocellular carcinoma cell line Hep G2.” Particle and Fibre Toxicology, vol. 10, no. 1, 2013, p. 27., doi:10.1186/1743-8977-10-27.
- Chu, Bryan, et al. “Graphene-Enhanced Environmentally-Benign Cutting Fluids for High-Performance Micro-Machining Applications.” Journal of Nanoscience and Nanotechnology, vol. 13, no. 8, Jan. 2013, pp. 5500–5504., doi:10.1166/jnn.2013.7538.
- Lammel, Tobias, and José M. Navas. “Graphene nanoplatelets spontaneously translocate into the cytosol and physically interact with cellular organelles in the fish cell line PLHC-1.” Aquatic Toxicology, vol. 150, 2014, pp. 55–65., doi:10.1016/j.aquatox.2014.02.016.
- Lammel, Tobias, et al. “Potentiating effect of graphene nanomaterials on aromatic environmental pollutant-Induced cytochrome P450 1A expression in the topminnow fish hepatoma cell line PLHC-1.” Environmental Toxicology, vol. 30, no. 10, May 2014, pp. 1192–1204., doi:10.1002/tox.21991.
- Liu, Zhenbao, et al. “Intracellular Detection of ATP Using an Aptamer Beacon Covalently Linked to Graphene Oxide Resisting Nonspecific Probe Displacement.” Analytical Chemistry, vol. 86, no. 24, 2014, pp. 12229–12235., doi:10.1021/ac503358m.
- Robertson, Neil M., et al. “Monitoring the Multitask Mechanism of DNase I Activity Using Graphene Nanoassemblies.” Bioconjugate Chemistry, vol. 26, no. 4, Oct. 2015, pp. 735–745., doi:10.1021/acs.bioconjchem.5b00067.
- Balcioglu, Mustafa, et al. “Doxorubicin loading on graphene oxide, iron oxide and gold nanoparticle hybrid.” Journal of Materials Chemistry B, vol. 1, no. 45, 2013, p. 6187., doi:10.1039/c3tb20992j.
- Rana, Muhit, et al. “Nano-Graphene oxide as a novel platform for monitoring the effect of LNA modification on nucleic acid interactions.” The Analyst, vol. 139, no. 4, 2014, pp. 714–720., doi:10.1039/c3an02066e.
- Kaplan, Amir, et al. “Structures Self-Assembled from Anionic Graphene and Cationic Manganese Porphyrin: Characterization and Application in Artificial Photosynthesis.” European Journal of Inorganic Chemistry, vol. 2014, no. 13, 2014, pp. 2288–2295., doi:10.1002/ejic.201400054.
- Balcioglu, Mustafa, et al. “DNA-Length-Dependent Quenching of Fluorescently Labeled Iron Oxide Nanoparticles with Gold, Graphene Oxide and MoS2 Nanostructures.” ACS Applied Materials & Interfaces, vol. 6, no. 15, 2014, pp. 12100–12110., doi:10.1021/am503553h.
- Balcioglu, Mustafa, et al. “Smart-Polymer-Functionalized Graphene Nanodevices for Thermo-Switch-Controlled Biodetection.” ACS Biomaterials Science & Engineering, vol. 1, no. 1, Dec. 2015, pp. 27–36., doi:10.1021/ab500029h.
- Lu, Chang, et al. “Covalent linking DNA to graphene oxide and its comparison with physisorbed probes for Hg 2 detection.” Biosensors and Bioelectronics, vol. 79, 2016, pp. 244–250., doi:10.1016/j.bios.2015.12.043.
- Mauro, Nicolò, et al. “Biotin-Containing Reduced Graphene Oxide-Based Nanosystem as a Multieffect Anticancer Agent: Combining Hyperthermia with Targeted Chemotherapy.” Biomacromolecules, vol. 16, no. 9, May 2015, pp. 2766–2775., doi:10.1021/acs.biomac.5b00705.
- Robertson, Neil M., et al. “Discriminating a Single Nucleotide Difference for Enhanced miRNA Detection Using Tunable Graphene and Oligonucleotide Nanodevices.” Langmuir, vol. 31, no. 36, Feb. 2015, pp. 9943–9952., doi:10.1021/acs.langmuir.5b02026.
- Robertson, Neil M., et al. “Unlocked Nucleic Acids for miRNA detection using two dimensional nano-Graphene oxide.” Biosensors and Bioelectronics, vol. 89, 2017, pp. 551–557., doi:10.1016/j.bios.2016.02.058.
- Lu, Chang, et al. “Comparison of Graphene Oxide and Reduced Graphene Oxide for DNA Adsorption and Sensing.” Langmuir, vol. 32, no. 41, June 2016, pp. 10776–10783., doi:10.1021/acs.langmuir.6b03032.
- Hizir, Mustafa Salih, et al. “Universal sensor array for highly selective system identification using two-Dimensional nanoparticles.” Chemical Science, vol. 8, no. 8, 2017, pp. 5735–5745., doi:10.1039/c7sc01522d.
- Qin, Ailin, et al. “Precipitation of PEG/Carboxyl-Modified Gold Nanoparticles with Magnesium Pyrophosphate: A New Platform for Real-Time Monitoring of Loop-Mediated Isothermal Amplification.” ACS Applied Materials & Interfaces, vol. 9, no. 12, 2017, pp. 10472–10480., doi:10.1021/acsami.7b00046.
- Mehmeti, Valbonë, and Fetah I. Podvorica. "Experimental and Theoretical Studies on Corrosion Inhibition of Niobium and Tantalum Surfaces by Carboxylated Graphene Oxide." Materials 11, no. 6 (2018): 893.
- Hizir, Mustafa Salih, Nidhi Nandu, and Mehmet V. Yigit. "Homologous miRNA analyses using a combinatorial nanosensor array with two-dimensional nanoparticles." Analytical chemistry 90, no. 10 (2018): 6300-6306.
- Nandu, Nidhi, Mustafa Salih Hizir, and Mehmet V. Yigit. "Systematic investigation of two-dimensional DNA nanoassemblies for construction of a nonspecific sensor array." Langmuir 34, no. 49 (2018): 14983-14992.
|
-
FAQ
1. What is the synthesis method of this product?
The synthesis method is made of small organic molecules (bottom-up method).
Research Citations of ACS Material Products
- Li, Changzheng, and Yanan Yue. “Fluorescence spectroscopy of graphene quantum dots: temperature effect at different excitation wavelengths.” Nanotechnology, vol. 25, no. 43, Sept. 2014, p. 435703., doi:10.1088/0957-4484/25/43/435703.
- Bhatnagar, Deepika, et al. “Graphene quantum dots FRET based sensor for early detection of heart attack in human.” Biosensors and Bioelectronics, vol. 79, 2016, pp. 495–499., doi:10.1016/j.bios.2015.12.083.
- Liu, Yang, et al. “Electro-Optical switching of liquid crystals sandwiched between ion-Beam-Spurted graphene quantum dots-Doped PEDOT:PSS composite layers.” Optics Express, vol. 23, no. 26, 2015, p. 34071., doi:10.1364/oe.23.034071.
- Nieto, Andy, et al. “Graphene reinforced metal and ceramic matrix composites: a review.” International Materials Reviews, vol. 62, no. 5, 2016, pp. 241–302., doi:10.1080/09506608.2016.1219481.
- Bok, Chang Han, et al. “Operating mechanisms of highly-Reproducible write-Once-Read-Many-Times memory devices based on graphene quantum dot:Poly(Methyl silsesquioxane) nanocomposites.” Applied Physics Letters, vol. 110, no. 1, Apr. 2017, p. 013301., doi:10.1063/1.4973358.
- Kim, Do Hyeong, et al. “Highly-Reproducible nonvolatile memristive devices based on polyvinylpyrrolidone: Graphene quantum-Dot nanocomposites.” Organic Electronics, vol. 51, 2017, pp. 156–161., doi:10.1016/j.orgel.2017.09.005.
- Gupta, Shagun, et al. “Ultrasensitive transglutaminase based nanosensor for early detection of celiac disease in human.” International Journal of Biological Macromolecules, vol. 105, 2017, pp. 905–911., doi:10.1016/j.ijbiomac.2017.07.126.
- Ooi, Poh Choon, et al. “Reduced graphene oxide preparation and its applications in solution-Processed write-Once-Read-Many-Times graphene-Based memory device.” Carbon, vol. 124, 2017, pp. 547–554., doi:10.1016/j.carbon.2017.09.004.
- Choi, Hwan Young, et al. “Organic electronic synapses with pinched hystereses based on graphene quantum-Dot nanocomposites.” NPG Asia Materials, vol. 9, no. 7, 2017, doi:10.1038/am.2017.133.
- Ooi, Poh Choon, et al. “Fabrication of transparent bistable switching memory device using plasmapolymerized hexamethyldisiloxane layers with embedded graphene quantum dots.” Thin Solid Films, vol. 645, 2018, pp. 45–50., doi:10.1016/j.tsf.2017.10.044.
- Abidin, Shariffah Nur Jannah Syed Zainol, et al. “Electropolymerization of poly(3,4-Ethylenedioxythiophene) onto polyvinyl alcohol-Graphene quantum dot-Cobalt oxide nanofiber composite for high-Performance supercapacitor.” Electrochimica Acta, vol. 261, 2018, pp. 548–556., doi:10.1016/j.electacta.2017.12.168.
- Wang, Changhong, et al. “Computing: Memristive Devices with Highly Repeatable Analog States Boosted by Graphene Quantum Dots.” Small, vol. 13, no. 20, 2017, doi:10.1002/smll.201770110.
- Bakar, Elyani Abu, Mohd Ambri Mohamed, Poh Choon Ooi, MF Mohd Razip Wee, Chang Fu Dee, and Burhanuddin Yeop Majlis. "Fabrication of indium-tin-oxide free, all-solution-processed flexible nanogenerator device using nanocomposite of barium titanate and graphene quantum dots in polyvinylidene fluoride polymer matrix." Organic Electronics 61 (2018): 289-295.
- Ooi, Poh Choon, MF Mohd Razip Wee, Chang Fu Dee, Chi Chin Yap, Muhammad Mat Salleh, and Burhanuddin Yeop Majlis. "Fabrication of transparent bistable switching memory device using plasmapolymerized hexamethyldisiloxane layers with embedded graphene quantum dots." Thin Solid Films 645 (2018): 45-50.
- Sung, Sihyun, Chaoxing Wu, Hyun Soo Jung, and Tae Whan Kim. "Highly-stable write-once-read-many-times switching behaviors of 1D–1R memristive devices based on graphene quantum dot nanocomposites." Scientific reports 8, no. 1 (2018): 12081.
- Sarkar, Kalyan Jyoti, K. Sarkar, B. Pal, and P. Banerji. "Graphene quantum dots as charge trap elements for nonvolatile flash memory." Journal of Physics and Chemistry of Solids 122 (2018): 137-142.
|
-
FAQ
1. What is the synthesis method of this product?
The synthesis method is made of small organic molecules (bottom-up method).
Research Citations of ACS Material Products
- Li, Changzheng, and Yanan Yue. “Fluorescence spectroscopy of graphene quantum dots: temperature effect at different excitation wavelengths.” Nanotechnology, vol. 25, no. 43, Sept. 2014, p. 435703., doi:10.1088/0957-4484/25/43/435703.
- Bhatnagar, Deepika, et al. “Graphene quantum dots FRET based sensor for early detection of heart attack in human.” Biosensors and Bioelectronics, vol. 79, 2016, pp. 495–499., doi:10.1016/j.bios.2015.12.083.
- Liu, Yang, et al. “Electro-Optical switching of liquid crystals sandwiched between ion-Beam-Spurted graphene quantum dots-Doped PEDOT:PSS composite layers.” Optics Express, vol. 23, no. 26, 2015, p. 34071., doi:10.1364/oe.23.034071.
- Nieto, Andy, et al. “Graphene reinforced metal and ceramic matrix composites: a review.” International Materials Reviews, vol. 62, no. 5, 2016, pp. 241–302., doi:10.1080/09506608.2016.1219481.
- Bok, Chang Han, et al. “Operating mechanisms of highly-Reproducible write-Once-Read-Many-Times memory devices based on graphene quantum dot:Poly(Methyl silsesquioxane) nanocomposites.” Applied Physics Letters, vol. 110, no. 1, Apr. 2017, p. 013301., doi:10.1063/1.4973358.
- Kim, Do Hyeong, et al. “Highly-Reproducible nonvolatile memristive devices based on polyvinylpyrrolidone: Graphene quantum-Dot nanocomposites.” Organic Electronics, vol. 51, 2017, pp. 156–161., doi:10.1016/j.orgel.2017.09.005.
- Gupta, Shagun, et al. “Ultrasensitive transglutaminase based nanosensor for early detection of celiac disease in human.” International Journal of Biological Macromolecules, vol. 105, 2017, pp. 905–911., doi:10.1016/j.ijbiomac.2017.07.126.
- Ooi, Poh Choon, et al. “Reduced graphene oxide preparation and its applications in solution-Processed write-Once-Read-Many-Times graphene-Based memory device.” Carbon, vol. 124, 2017, pp. 547–554., doi:10.1016/j.carbon.2017.09.004.
- Choi, Hwan Young, et al. “Organic electronic synapses with pinched hystereses based on graphene quantum-Dot nanocomposites.” NPG Asia Materials, vol. 9, no. 7, 2017, doi:10.1038/am.2017.133.
- Ooi, Poh Choon, et al. “Fabrication of transparent bistable switching memory device using plasmapolymerized hexamethyldisiloxane layers with embedded graphene quantum dots.” Thin Solid Films, vol. 645, 2018, pp. 45–50., doi:10.1016/j.tsf.2017.10.044.
- Abidin, Shariffah Nur Jannah Syed Zainol, et al. “Electropolymerization of poly(3,4-Ethylenedioxythiophene) onto polyvinyl alcohol-Graphene quantum dot-Cobalt oxide nanofiber composite for high-Performance supercapacitor.” Electrochimica Acta, vol. 261, 2018, pp. 548–556., doi:10.1016/j.electacta.2017.12.168.
- Wang, Changhong, et al. “Computing: Memristive Devices with Highly Repeatable Analog States Boosted by Graphene Quantum Dots.” Small, vol. 13, no. 20, 2017, doi:10.1002/smll.201770110.
- Bakar, Elyani Abu, Mohd Ambri Mohamed, Poh Choon Ooi, MF Mohd Razip Wee, Chang Fu Dee, and Burhanuddin Yeop Majlis. "Fabrication of indium-tin-oxide free, all-solution-processed flexible nanogenerator device using nanocomposite of barium titanate and graphene quantum dots in polyvinylidene fluoride polymer matrix." Organic Electronics 61 (2018): 289-295.
- Ooi, Poh Choon, MF Mohd Razip Wee, Chang Fu Dee, Chi Chin Yap, Muhammad Mat Salleh, and Burhanuddin Yeop Majlis. "Fabrication of transparent bistable switching memory device using plasmapolymerized hexamethyldisiloxane layers with embedded graphene quantum dots." Thin Solid Films 645 (2018): 45-50.
- Sung, Sihyun, Chaoxing Wu, Hyun Soo Jung, and Tae Whan Kim. "Highly-stable write-once-read-many-times switching behaviors of 1D–1R memristive devices based on graphene quantum dot nanocomposites." Scientific reports 8, no. 1 (2018): 12081.
- Sarkar, Kalyan Jyoti, K. Sarkar, B. Pal, and P. Banerji. "Graphene quantum dots as charge trap elements for nonvolatile flash memory." Journal of Physics and Chemistry of Solids 122 (2018): 137-142.
|
-
Research Citations of ACS Material Products
- Li, Changzheng, and Yanan Yue. “Fluorescence spectroscopy of graphene quantum dots: temperature effect at different excitation wavelengths.” Nanotechnology, vol. 25, no. 43, Sept. 2014, p. 435703., doi:10.1088/0957-4484/25/43/435703.
- Bhatnagar, Deepika, et al. “Graphene quantum dots FRET based sensor for early detection of heart attack in human.” Biosensors and Bioelectronics, vol. 79, 2016, pp. 495–499., doi:10.1016/j.bios.2015.12.083.
- Liu, Yang, et al. “Electro-Optical switching of liquid crystals sandwiched between ion-Beam-Spurted graphene quantum dots-Doped PEDOT:PSS composite layers.” Optics Express, vol. 23, no. 26, 2015, p. 34071., doi:10.1364/oe.23.034071.
- Bhatnagar, Deepika, et al. “Ultrasensitive cardiac troponin I antibody based nanohybrid sensor for rapid detection of human heart attack.” International Journal of Biological Macromolecules, vol. 95, 2017, pp. 505–510., doi:10.1016/j.ijbiomac.2016.11.037.
- Nieto, Andy, et al. “Graphene reinforced metal and ceramic matrix composites: a review.” International Materials Reviews, vol. 62, no. 5, 2016, pp. 241–302., doi:10.1080/09506608.2016.1219481.
- Bok, Chang Han, et al. “Operating mechanisms of highly-Reproducible write-Once-Read-Many-Times memory devices based on graphene quantum dot:Poly(Methyl silsesquioxane) nanocomposites.” Applied Physics Letters, vol. 110, no. 1, Apr. 2017, p. 013301., doi:10.1063/1.4973358.
- Kim, Do Hyeong, et al. “Highly-Reproducible nonvolatile memristive devices based on polyvinylpyrrolidone: Graphene quantum-Dot nanocomposites.” Organic Electronics, vol. 51, 2017, pp. 156–161., doi:10.1016/j.orgel.2017.09.005.
- Gupta, Shagun, et al. “Ultrasensitive transglutaminase based nanosensor for early detection of celiac disease in human.” International Journal of Biological Macromolecules, vol. 105, 2017, pp. 905–911., doi:10.1016/j.ijbiomac.2017.07.126.
- Ooi, Poh Choon, et al. “Reduced graphene oxide preparation and its applications in solution-Processed write-Once-Read-Many-Times graphene-Based memory device.” Carbon, vol. 124, 2017, pp. 547–554., doi:10.1016/j.carbon.2017.09.004.
- Choi, Hwan Young, et al. “Organic electronic synapses with pinched hystereses based on graphene quantum-Dot nanocomposites.” NPG Asia Materials, vol. 9, no. 7, 2017, doi:10.1038/am.2017.133.
- Ooi, Poh Choon, et al. “Fabrication of transparent bistable switching memory device using plasmapolymerized hexamethyldisiloxane layers with embedded graphene quantum dots.” Thin Solid Films, vol. 645, 2018, pp. 45–50., doi:10.1016/j.tsf.2017.10.044.
- Wang, Changhong, et al. “Computing: Memristive Devices with Highly Repeatable Analog States Boosted by Graphene Quantum Dots.” Small, vol. 13, no. 20, 2017, doi:10.1002/smll.201770110.
- Bakar, Elyani Abu, Mohd Ambri Mohamed, Poh Choon Ooi, MF Mohd Razip Wee, Chang Fu Dee, and Burhanuddin Yeop Majlis. "Fabrication of indium-tin-oxide free, all-solution-processed flexible nanogenerator device using nanocomposite of barium titanate and graphene quantum dots in polyvinylidene fluoride polymer matrix." Organic Electronics 61 (2018): 289-295.
- Ooi, Poh Choon, MF Mohd Razip Wee, Chang Fu Dee, Chi Chin Yap, Muhammad Mat Salleh, and Burhanuddin Yeop Majlis. "Fabrication of transparent bistable switching memory device using plasmapolymerized hexamethyldisiloxane layers with embedded graphene quantum dots." Thin Solid Films 645 (2018): 45-50.
- Sung, Sihyun, Chaoxing Wu, Hyun Soo Jung, and Tae Whan Kim. "Highly-stable write-once-read-many-times switching behaviors of 1D–1R memristive devices based on graphene quantum dot nanocomposites." Scientific reports 8, no. 1 (2018): 12081.
|
-
Research Citations of ACS Material Products
1. Bailey, Alta C. "In-Situ Densification of Metal Binder Jet Printed Components via Nanoparticles." PhD diss., North Carolina Agricultural and Technical State University, 2018.
|
-
Research Citations of ACS Material Products
- Hwang, Chihyun, et al. “All-in-One assembly based on 3D-Intertangled and cross-Jointed architectures of Si/Cu 1D-Nanowires for lithium ion batteries.” Scientific Reports, vol. 5, no. 1, 2015, doi:10.1038/srep08623.
|
-
Research Citations of ACS Material Products
- Hwang, Chihyun, et al. “All-in-One assembly based on 3D-Intertangled and cross-Jointed architectures of Si/Cu 1D-Nanowires for lithium ion batteries.” Scientific Reports, vol. 5, no. 1, 2015, doi:10.1038/srep08623.
|
-
FAQ
1. What's the difference between CVD graphene on copper foil and CVD graphene on copper foil (graphene factory)?
Our CVD Graphene on Copper Foil (Graphene Factory) product is designed for large size wafer transfers (up to 6x6in) and currently only the monolayer type is available. As the size increases there are likely to be more defects as compared to our standard CVD Graphene on Cu Foil. In general, our standard CVD Graphene on Copper Foil has better quality and the size is available up to 6inch x 4inch. This one has better monolayer graphene coverage because it is easier to control for smaller sizes. Both products are prepared by the CVD method, but we use a different set of equipment, so we name the products differently to make that distinction. You may choose whichever works best for your application.
2. What is the step by step transfer process of CVD graphene on copper foil onto my own substrates?
The PMMA method is also explained on the blogpot and you may check out/use whichever method that you see fit for your application.
3. How much N-doped CVD graphene on copper foil would be?
Our CVD Graphene on Copper Foil product is not N-doped graphene.
4. What is the graphene layer thickness for each layers?
The graphene thickness for the 1 Layer is about 1nm. For 2 Layer it is about 1.3nm. For 3-5 Layers, it's about 1.6nm-2.4nm. For 6-8 Layers, it's about 2.7nm-3.4nm.
Research Citations of ACS Material Products
- Chen, Ruiyi, et al. “Co-Percolating Graphene-Wrapped Silver Nanowire Network for High Performance, Highly Stable, Transparent Conducting Electrodes.” Advanced Functional Materials, vol. 23, no. 41, 2013, pp. 5150–5158., doi:10.1002/adfm.201300124.
- O’Hern, Sean C., et al. “Selective Molecular Transport through Intrinsic Defects in a Single Layer of CVD Graphene.” ACS Nano, vol. 6, no. 11, Sept. 2012, pp. 10130–10138., doi:10.1021/nn303869m.
- Yoo, Jae-Hyuck, et al. “Graphene folds by femtosecond laser ablation.” Applied Physics Letters, vol. 100, no. 23, Apr. 2012, p. 233124., doi:10.1063/1.4724213.
- Longchamp, Jean-Nicolas, et al. “Low-Energy electron transmission imaging of clusters on free-Standing graphene.” Applied Physics Letters, vol. 101, no. 11, Oct. 2012, p. 113117., doi:10.1063/1.4752717.
- Chen, Xu-Dong, et al. “High-Quality and efficient transfer of large-Area graphene films onto different substrates.” Carbon, vol. 56, May 2013, pp. 271–278., doi:10.1016/j.carbon.2013.01.011.
- Ye, Qing., et al. “Polarization-Dependent optical absorption of graphene under total internal reflection.” Applied Physics Letters, vol. 102, no. 2, doi:10.1063/1.4776694.
- Longchamp, Jean-Nicolas, et al. “Ultraclean freestanding graphene by platinum-Metal catalysis.” Journal of Vacuum Science & Technology B, Nanotechnology and Microelectronics: Materials, Processing, Measurement, and Phenomena, vol. 31, no. 2, 2013, p. 020605., doi:10.1116/1.4793746.
- Wang, Yung Yu, and Peter J. Burke. “A large-Area and contamination-Free graphene transistor for liquid-Gated sensing applications.” Applied Physics Letters, vol. 103, no. 5, 2013, p. 052103., doi:10.1063/1.4816764.
- Wang, Peng, et al. “Accurate layers determination of graphene on transparent substrate based on polarization-Sensitive absorption effect.” Applied Physics Letters, vol. 103, no. 18, 2013, p. 181902., doi:10.1063/1.4827812.
- Zhou, Rong, et al. “Large-Energy, narrow-Bandwidth laser pulse at 1645 nm in a diode-Pumped Er:YAG solid-State laser passively Q-Switched by a monolayer graphene saturable absorber.” Applied Optics, vol. 53, no. 2, Sept. 2014, p. 254., doi:10.1364/ao.53.000254.
- Choi, Duyoung, et al. “Nanopatterned Graphene Field Effect Transistor Fabricated Using Block Co-Polymer Lithography.” Materials Research Letters, vol. 2, no. 3, Sept. 2014, pp. 131–139., doi:10.1080/21663831.2013.876676.
- Srisonphan, Siwapon, et al. “Space charge neutralization by electron-Transparent suspended graphene.” Scientific Reports, vol. 4, no. 1, 2014, doi:10.1038/srep03764.
- Joiner, C. A., et al. “Cleaning graphene with a titanium sacrificial layer.” Applied Physics Letters, vol. 104, no. 22, Feb. 2014, p. 223109., doi:10.1063/1.4881886.
- Roy, T., et al. “Tunneling characteristics in chemical vapor deposited graphene–hexagonal boron nitride–graphene junctions.” Applied Physics Letters, vol. 104, no. 12, 2014, p. 123506., doi:10.1063/1.4870073.
- Burke, Peter J. “Charging the Quantum Capacitance of Graphene with a Single Biological Ion Channel.” Biophysical Journal, vol. 106, no. 2, 2014, doi:10.1016/j.bpj.2013.11.2796.
- Li, Peining, et al. “Graphene-Enhanced Infrared Near-Field Microscopy.” Nano Letters, vol. 14, no. 8, 2014, pp. 4400–4405., doi:10.1021/nl501376a.
- Boutilier, Michael S. H., et al. “Implications of Permeation through Intrinsic Defects in Graphene on the Design of Defect-Tolerant Membranes for Gas Separation.” ACS Nano, vol. 8, no. 1, Mar. 2014, pp. 841–849., doi:10.1021/nn405537u.
- Lee, Jiye, et al. “Switching Individual Quantum Dot Emission through Electrically Controlling Resonant Energy Transfer to Graphene.” Nano Letters, vol. 14, no. 12, 2014, pp. 7115–7119., doi:10.1021/nl503587z.
- Srisonphan, Siwapon, and Komsan Hongesombut. “Tuning the ballistic electron transport of spatial graphene–metal sandwich electrode on a vacuum-Silicon-Based device.” RSC Advances, vol. 5, no. 3, 2015, pp. 2032–2037., doi:10.1039/c4ra09503k.
- Hui, Fei, et al. “Mechanical properties of locally oxidized graphene electrodes.” Archive of Applied Mechanics, vol. 85, no. 3, 2014, pp. 339–345., doi:10.1007/s00419-014-0957-4.
- Fujimoto, A, et al. “Negative magnetoresistance in Ti-Cleaned single-Layer graphene.” Journal of Physics: Conference Series, vol. 603, 2015, p. 012021., doi:10.1088/1742-6596/603/1/012021.
- Thareja, Vrinda, et al. “Electrically Tunable Coherent Optical Absorption in Graphene with Ion Gel.” Nano Letters, vol. 15, no. 3, 2015, pp. 1570–1576., doi:10.1021/nl503431d.
- Ye, X. H., et al. “Corrosion resistance of graphene directly and locally grown on bulk nickel substrate by laser irradiation.” RSC Advances, vol. 5, no. 45, 2015, pp. 35384–35390., doi:10.1039/c5ra01267h.
- Horvath, Cameron . “Fabrication and Characterization of Edge-Conformed Graphene-Silicon Waveguides.” IEEE Photonics Technology Letters, vol. 27, no. 6, 6 Jan. 2015, pp. 585–587., doi:10.1109/LPT.2014.2385757.
- Ye, Qing. “Polarization-Dependent optical absorption of graphene under total internal reflection.” Applied Physics Letters, vol. 102, no. 2, Jan. 2013, doi:10.1063/1.4776694.
- Zheng, Guanpeng, et al. “Improved Transfer Quality of CVD-Grown Graphene by Ultrasonic Processing of Target Substrates: Applications for Ultra-Fast Laser Photonics.” ACS Applied Materials & Interfaces, vol. 5, no. 20, Sept. 2013, pp. 10288–10293., doi:10.1021/am403205v.
- Zeng, Yong, et al. “Investigate the interface structure and growth mechanism of high quality ZnO films grown on multilayer graphene layers.” Applied Surface Science, vol. 301, 2014, pp. 391–395., doi:10.1016/j.apsusc.2014.02.088.
- Tang, Pinghua, et al. “Stable high-Energy Q-Switched resonantly diode-Pumped Er:YAG laser at 1645 nm.” OSA Publishing, vol. 53, no. 32, 2014, pp. 7773–7777., doi:10.1364/AO.53.007773.
- Watanabe, Hiroshi, et al. “Layer number dependence of carrier lifetime in graphenes observed using time-Resolved mid-Infrared luminescence.” Chemical Physics Letters, vol. 637, 2015, pp. 58–62., doi:10.1016/j.cplett.2015.07.046.
- He, Yingbo, et al. “Strongly enhanced Raman scattering of graphene by a single gold nanorod.” Applied Physics Letters, vol. 107, no. 5, Mar. 2015, p. 053104., doi:10.1063/1.4927759.
- Choi, Duyoung, et al. “Uniformly Nanopatterned Graphene Field-Effect Transistors with Enhanced Properties.” Nanoscale Research Letters, vol. 10, no. 1, Nov. 2015, doi:10.1186/s11671-015-0976-2.
- Miao, Lili, et al. “Broadband ultrafast nonlinear optical response of few-Layers graphene: toward the mid-Infrared regime.” Photonics Research, vol. 3, no. 5, 2015, p. 214., doi:10.1364/prj.3.000214.
- Hu, Jianchen, et al. “Improvement of the electrical contact resistance at rough interfaces using two dimensional materials.” Journal of Applied Physics, vol. 118, no. 21, July 2015, p. 215301., doi:10.1063/1.4936366.
- Jain, Tarun, et al. “Heterogeneous sub-Continuum ionic transport in statistically isolated graphene nanopores.” Nature Nanotechnology, vol. 10, no. 12, May 2015, pp. 1053–1057., doi:10.1038/nnano.2015.222.
- Srisonphan, Siwapon, and Komsan Hongesombut. “Tuning the ballistic electron transport of spatial graphene–metal sandwich electrode on a vacuum-Silicon-Based device.” RSC Advances, vol. 5, no. 3, 2015, pp. 2032–2037., doi:10.1039/c4ra09503k.
- Liu, Xiangjiang, et al. “Compact Shielding of Graphene Monolayer Leads to Extraordinary SERS-Active Substrate with Large-Area Uniformity and Long-Term Stability.” Scientific Reports, vol. 5, no. 1, 2015, doi:10.1038/srep17167.
- Hui, Fei, et al. “Moving graphene devices from lab to market: advanced graphene-Coated nanoprobes.” Nanoscale, vol. 8, no. 16, 2016, pp. 8466–8473., doi:10.1039/c5nr06235g.
- Ye, Xiaohui, et al. “Protecting carbon steel from corrosion by laser in situ grown graphene films.” Carbon, vol. 94, 2015, pp. 326–334., doi:10.1016/j.carbon.2015.06.080.
- Wu, Man, et al. “Wavelength switchable graphene Q-Switched fiber laser with cascaded fiber Bragg gratings.” Optics Communications, vol. 368, 2016, pp. 81–85., doi:10.1016/j.optcom.2016.01.069.
- Mackin, Charles, and Tomás Palacios. “Large-Scale sensor systems based on graphene electrolyte-Gated field-Effect transistors.” The Analyst, vol. 141, no. 9, 2016, pp. 2704–2711., doi:10.1039/c5an02328a.
- Politou, Maria, et al. “Multi-Layer graphene interconnect.” IEEE Xplore Digital Library , 11 July 2016, doi:10.1109/IITC-AMC.2016.7507731.
- Cao, Zhengmin, et al. “Nano-Gap between a gold tip and nanorod for polarization dependent surface enhanced Raman scattering.” Applied Physics Letters, vol. 109, no. 23, May 2016, p. 233103., doi:10.1063/1.4971832.
- Deng, Xiangquan, et al. “Terahertz-Induced photothermoelectric response in graphene-Metal contact structures.” Journal of Physics D: Applied Physics, vol. 49, no. 42, 2016, p. 425101., doi:10.1088/0022-3727/49/42/425101.
- Guo, Chu‐Cai, et al. “Experimental Demonstration of Total Absorption over 99% in the Near Infrared for Monolayer‐Graphene‐Based Subwavelength Structures.” Advanced Optical Materials, 1 Sept. 2016, onlinelibrary.wiley.com/doi/10.1002/adom.201600481/full.
- Hu, Jianbo, et al. “Rippling ultrafast dynamics of suspended 2D monolayers, graphene.” Proceedings of the National Academy of Sciences, vol. 113, no. 43, Oct. 2016, doi:10.1073/pnas.1613818113.
- Li, Cheng, et al. “Measurement of the Adhesion Energy of Pressurized Graphene Diaphragm Using Optical Fiber Fabry–Perot Interference.” IEEE Sensors Journal, vol. 16, no. 10, 2016, pp. 3664–3669., doi:10.1109/jsen.2016.2536783.
- Yuan, Jun, et al. “Modulation of far-Infrared light transmission by graphene-Silicon Schottky junction.” Optical Materials Express, vol. 6, no. 12, 2016, p. 3908., doi:10.1364/ome.6.003908.
- Li, Cheng, et al. “Measurement of thermal expansion coefficient of graphene diaphragm using optical fiber Fabry–Perot interference.” Measurement Science and Technology, vol. 27, no. 7, 2016, p. 075102., doi:10.1088/0957-0233/27/7/075102.
- Kafiah, Feras M., et al. “Monolayer graphene transfer onto polypropylene and polyvinylidenedifluoride microfiltration membranes for water desalination.” Desalination, vol. 388, 2016, pp. 29–37., doi:10.1016/j.desal.2016.02.027.
- Wu, Xiangyu. “Doping of graphene for the application in nano-Interconnect.” Microelectronic Engineering, vol. 167, 5 Jan. 2017, pp. 42–46., doi:10.1016/j.mee.2016.10.013.
- Liu, Siyang, et al. “Atomic emission spectroscopy of electrically triggered exploding nanoparticle analytes on graphene/SiO2/Si substrate.” Journal of Vacuum Science & Technology B, Nanotechnology and Microelectronics: Materials, Processing, Measurement, and Phenomena, vol. 34, no. 6, doi:10.1116/1.4964819.
- Sattler, Klaus D. Carbon nanomaterials sourcebook. Vol. 1, CRC Press, 2016.
- Politou, Maria. “Evaluation of multilayer graphene for advanced interconnects.” Microelectronic Engineering, vol. 167, 5 Jan. 2017, pp. 1–5., doi:10.1016/j.mee.2016.09.011.
- Hwang, Michael T., et al. “Highly specific SNP detection using 2D graphene electronics and DNA strand displacement.” Proceedings of the National Academy of Sciences, vol. 113, no. 26, 2016, pp. 7088–7093., doi:10.1073/pnas.1603753113.
- Nieto, Andy, et al. “Graphene reinforced metal and ceramic matrix composites: a review.” International Materials Reviews, vol. 62, no. 5, 2017, pp. 241–302., doi:10.1080/09506608.2016.1219481.
- Khorasaninejad, M., et al. “Highly Enhanced Raman Scattering of Graphene using Plasmonic Nano-Structure.” Scientific Reports, vol. 3, no. 1, 2013, doi:10.1038/srep02936.
- Kunz, Daniel A., et al. “Space-Resolved In-Plane Moduli of Graphene Oxide and Chemically Derived Graphene Applying a Simple Wrinkling Procedure (Adv. Mater. 9/2013).” Advanced Materials, vol. 25, no. 9, Apr. 2013, pp. 1336–1336., doi:10.1002/adma.201370060.
- Peng, Shuhua, et al. “Microwetting of Supported Graphene on Hydrophobic Surfaces Revealed by Polymerized Interfacial Femtodroplets.” Langmuir, vol. 30, no. 33, 2014, pp. 10043–10049., doi:10.1021/la5022774.
- Luo, Wen, et al. “Single-Layer Graphene as an Effective Mediator of the Metal–Support Interaction.” The Journal of Physical Chemistry Letters, vol. 5, no. 11, 2014, pp. 1837–1844., doi:10.1021/jz500425j.
- Suemoto, Tohru, et al. “Thickness dependent hot-Phonon effects observed by femtosecond mid-Infrared luminescence in graphene.” 19th International Conference on Ultrafast Phenomena, 2014, doi:10.1364/up.2014.07.mon.p1.32.
- Zhou, Haosen, et al. "Influence of CO2 On the Stability of Discharge Performance for Li-Air Battery with Hybrid Electrolyte Based On the Graphene Sheet”, Conference: 224th ECS Meeting. Oct. 2013, doi:10.1039/c3ra47258b.
- Thareja, Vrinda. “Electrically Tunable Optical Absorption in a Graphene-Based Salisbury Screen.” CORNELL UNIVERSITY LIBRARY, 7 Sept. 2014.
- Horvath, Cameron S. “Theses and Dissertations: Light Propagation and Photothermal Nonlinearity in Graphene-Si Waveguides.” University of Alberta Libraries, 26 Sept. 2013, doi:10.7939/R3ST1N .
- Li, Cheng, et al. “Interference characteristics in a Fabry–Perot cavity with graphene membrane for optical fiber pressure sensors.” Microsystem Technologies, vol. 21, no. 11, 2014, pp. 2297–2306., doi:10.1007/s00542-014-2333-2.
- Kou, Jun-Long, et al. “Platform for enhanced light–graphene interaction length and miniaturizing fiber stereo devices.” Optica, vol. 1, no. 5, 2014, p. 307., doi:10.1364/optica.1.000307.
- Karnik, Rohit. “Ionic and Molecular Transport Through Graphene Membranes.” Transport and Reactivity of Solutions in Confined Hydrosystems NATO Science for Peace and Security Series C: Environmental Security, Dec. 2013, pp. 95–102., doi:10.1007/978-94-007-7534-3_8.
- Warren, A.J, et al. “In vacuo X-Ray data collection from graphene-Wrapped protein crystals.” Acta Crystallographica Section D STRUCTURAL BIOLOGY, vol. 71, 1 Oct. 2015, pp. 2079–2088., doi:10.1107/S1399004715014194.
- Wen, Chenyu, et al. “Assessing kinetics of surface adsorption–desorption of gas molecules via electrical measurements.” Sensors and Actuators B: Chemical, vol. 223, 2016, pp. 791–798., doi:10.1016/j.snb.2015.10.019.
- Li, Cheng, et al. “Analyzing the applicability of miniature ultra-High sensitivity Fabry–Perot acoustic sensor using a nanothick graphene diaphragm.” Measurement Science and Technology, vol. 26, no. 8, Oct. 2015, p. 085101., doi:10.1088/0957-0233/26/8/085101.
- Li, Cheng, et al. “Analyzing the temperature sensitivity of Fabry-Perot sensor using multilayer graphene diaphragm.” OSA Publishing/ Optics Express, vol. 23, no. 21, 2015, pp. 27494–27502 ., doi:10.1364/OE.23.027494.
- Lau, K. Y., et al. “High Signal-To-Noise Ratio Q-Switching Erbium Doped Fiber Laser Pulse Emission Utilizing Single Layer Trivial Transfer Graphene Film Saturable Absorber.” Jurnal Teknologi, vol. 78, no. 3, 2016, doi:10.11113/jt.v78.7478.
- Zheng, Shijun, et al. “Acoustic charge transport induced by the surface acoustic wave in chemical doped graphene.” Applied Physics Letters, vol. 109, no. 18, 2016, p. 183110., doi:10.1063/1.4967192.
- Deng, Xiangquan, et al. “Terahertz-Induced photothermoelectric response in graphene-Metal contact structures.” Journal of Physics D: Applied Physics, vol. 49, no. 42, 2016, p. 425101., doi:10.1088/0022-3727/49/42/425101.
- Luo, Wen. “Graphene-Coated ZnO and SiO2 as Supports for CoO Nanoparticles with Enhanced Reducibility.” Chemphys, vol. 17, no. 19, 5 Oct. 2016, pp. 3055–3061., doi:10.1002/cphc.201600499.
- Lau, K.y., et al. “Passively mode-Locked soliton femtosecond pulses employing graphene saturable absorber.” Optics & Laser Technology, vol. 94, 2017, pp. 221–227., doi:10.1016/j.optlastec.2017.03.035.
- Fitri, Meika Aidil, et al. “Fabrication of TiO 2 -Graphene photocatalyst by direct chemical vapor deposition and its anti-Fouling property.” Materials Chemistry and Physics, vol. 198, 2017, pp. 42–48., doi:10.1016/j.matchemphys.2017.05.053.
- Li, Cheng, et al. “Nondestructive andin situdetermination of graphene layers using optical fiber Fabry–Perot interference.” Measurement Science and Technology, vol. 28, no. 2, Dec. 2017, p. 025206., doi:10.1088/1361-6501/aa54f8.
- Gao, Xiangyang, et al. “Measuring Graphene Adhesion on Silicon Substrate by Single and Dual Nanoparticle-Loaded Blister.” Advanced Materials Interfaces, vol. 4, no. 9, 2017, p. 1601023., doi:10.1002/admi.201601023.
- Tang, Xiaoduan, et al. “Five Orders of Magnitude Reduction in Energy Coupling across Corrugated Graphene/Substrate Interfaces.” ACS Applied Materials & Interfaces, vol. 6, no. 4, July 2014, pp. 2809–2818., doi:10.1021/am405388a.
- Morrow, W. K., et al. “(Invited) The Use of Graphene as a Solid State Diffusion Barrier.” ECS Transactions, vol. 61, no. 4, 2014, pp. 371–379., doi:10.1149/06104.0371ecst.
- Zheng, Haisheng, et al. “Ultrafine Pt nanoparticle induced doping/Strain of single layer graphene: experimental corroboration between conduction and Raman characteristics.” Journal of Materials Science: Materials in Electronics, vol. 26, no. 7, 2015, pp. 4746–4753., doi:10.1007/s10854-015-3043-y.
- Blinco , James P. “Spin-Coated carbon.” Chemical Science, no. 9, 12 June 2013, pp. 3411–3415., doi:10.1039/C3SC51396C.
- Zheng, H., et al. “Effect of Sub 1-Nm Pt Nanoparticle on the Conduction Properties of Graphene Based Field Effect Transistor.” ECS Transactions, vol. 61, no. 39, Jan. 2014, pp. 1–11., doi:10.1149/06139.0001ecst.
- Mckitterick, Christopher B., et al. “Electron-Phonon cooling in large monolayer graphene devices.” Physical Review B, vol. 93, no. 7, Apr. 2016, doi:10.1103/physrevb.93.075410
- Blinco , James P, et al. “Spin-Coated carbon.” Chemical Science, no. 9, 2013, pp. 3411–3415., doi:10.1039/C3SC51396C.
- Chan, Chun Yu. “Graphene based electrical biosensors for the detection of biomolecules.” The Hong Kong Polytechnic University, 2016.
- Pápa, Z., et al. “Spectroscopic ellipsometric investigation of graphene and thin carbon films from the point of view of depolarization effects.” Applied Surface Science, vol. 421, 2017, pp. 714–721., doi:10.1016/j.apsusc.2016.11.231.
- Hussain, Mushtaque, et al. “The improved piezoelectric properties of ZnO nanorods with oxygen plasma treatment on the single layer graphene coated polymer substrate.” Physica status solidi (a), vol. 211, no. 2, Oct. 2014, pp. 455–459., doi:10.1002/pssa.201300330.
- Kafiah, Feras, et al. “Synthesis of Graphene Based Membranes: Effect of Substrate Surface Properties on Monolayer Graphene Transfer.” Materials, vol. 10, no. 1, 2017, p. 86., doi:10.3390/ma10010086.
- Liang, Ji, et al. “Modulation of acousto-Electric current using a hybrid on-Chip AlN SAW/GFET device.” Applied Physics Letters, vol. 110, no. 24, Dec. 2017, p. 243504., doi:10.1063/1.4986481.
- Zhang, Yingjie, et al. "Electron transport in strain superlattices of graphene." Cornell University Library. 16 Mar. 2017.
- Yan, Xiao-Qing, et al. “Polarization dependence of graphene transient optical response: interplay between incident direction and anisotropic distribution of nonequilibrium carriers.” Journal of the Optical Society of America B, vol. 34, no. 1, 2016, p. 218., doi:10.1364/josab.34.000218.
- Lau, K.Y., et al. “Passively mode-Locked soliton femtosecond pulses employing graphene saturable absorber.” Optics & Laser Technology, vol. 94, 2017, pp. 221–227., doi:10.1016/j.optlastec.2017.03.035.
- Zhang, Yingjie, et al. “Electron transport in strain superlattices of graphene.” Research Gate, Mar. 2017.
- Peng, Shuhua, et al. “Microwetting of Supported Graphene on Hydrophobic Surfaces Revealed by Polymerized Interfacial Femtodroplets.” Langmuir, vol. 30, no. 33, 2014, pp. 10043–10049., doi:10.1021/la5022774.
- Horvath, Cameron, et al. “Edge-Conformed silicon-Graphene waveguides: Fabrication and measurements.” 11th International Conference on Group IV Photonics (GFP), 2014, doi:10.1109/group4.2014.6961953.
- Watanabe, Hiroshi, e al. “Femtosecond midi-Infrared luminescence with hot-Phonon effects in graphenes and graphite.”
- Hassan, Saad. Transparent conductive article.
- Liang, Ji, et al. “Manipulation of carriers in graphene using an on-Chip acoustic wave device.” 2017 IEEE International Ultrasonics Symposium (IUS), 2017, doi:10.1109/ultsym.2017.8092898.
- Luo, Wen, et al. “Interaction of bimetallic PtCo layers with bare and graphene-Covered ZnO(0001) supports.” Surface Science, vol. 669, 2018, pp. 64–70., doi:10.1016/j.susc.2017.11.001.
- Jiang, Wen-Shuai, et al. “Preparation of high-Quality graphene using triggered microwave reduction under an air atmosphere.” Journal of Materials Chemistry C, 2018, doi:10.1039/c7tc03957c.
- Dong, Nannan, et al. “Pressure and Temperature Sensor Based on Graphene Diaphragm and Fiber Bragg Gratings.” IEEE Photonics Technology Letters, 2017, pp. 1–1., doi:10.1109/lpt.2017.2786292.
- Huczko, Andrzej, et al. “Efficient one-Pot combustion synthesis of few-Layered graphene.” Physica status solidi (b), vol. 252, no. 11, 2015, pp. 2412–2417., doi:10.1002/pssb.201552233.
- Lu, Bingyu, et al. “Roughened cylindrical gold layer with curve graphene coating for enhanced sensitivity of fiber SPR sensor.” 2017 19th International Conference on Solid-State Sensors, Actuators and Microsystems (TRANSDUCERS), 2017, doi:10.1109/transducers.2017.7994461.
- Fitri, Meika Aidil, et al. “Fabrication of TiO 2 -Graphene photocatalyst by direct chemical vapor deposition and its anti-Fouling property.” Materials Chemistry and Physics, vol. 198, 2017, pp. 42–48., doi:10.1016/j.matchemphys.2017.05.053.
- Bezryadin, A, et al. “Large energy storage efficiency of the dielectric layer of graphene nanocapacitors.” Nanotechnology, vol. 28, no. 49, 2017, p. 495401., doi:10.1088/1361-6528/aa935c.
- Shi, Cheng, et al. “Metamaterial-Based graphene thermal emitter.” Nano Research, June 2017, doi:10.1007/s12274-017-1922-7.
- McCaffrey, Debra L., et al. “Mechanism of ion adsorption to aqueous interfaces: Graphene/Water vs. air/Water.” Proceedings of the National Academy of Sciences, vol. 114, no. 51, 2017, pp. 13369–13373., doi:10.1073/pnas.1702760114.
- Zhang, Qin, et al. “Negative differential resistance and hysteresis in graphene-Based organic light-Emitting devices.” Journal of Materials Chemistry C, The Royal Society of Chemistry, 1 Jan. 2018, pubs.rsc.org/en/Content/ArticleLanding/2017/TC/C7TC05148D#!
- Miskin, Marc Z., et al. “Graphene-Based bimorphs for micron-Sized, autonomous origami machines.” Proceedings of the National Academy of Sciences, vol. 115, no. 3, Feb. 2018, pp. 466–470., doi:10.1073/pnas.1712889115.
- Bukola, Saheed, et al. “Selective Proton/Deuteron Transport through Nafion|Graphene|Nafion Sandwich Structures at High Current Density.” Journal of the American Chemical Society, vol. 140, no. 5, 2018, pp. 1743–1752., doi:10.1021/jacs.7b10853.
- Brown, Morgan A., et al. “Graphene Biotransistor Interfaced with a Nitrifying Biofilm.” Environmental Science & Technology Letters, vol. 2, no. 4, Mar. 2015, pp. 118–122., doi:10.1021/acs.estlett.5b00025.
- Woo, Sung Oh, and Winfried Teizer. “The effect of electron induced hydrogenation of graphene on its electrical transport properties.” Applied Physics Letters, vol. 103, no. 4, 2013, p. 041603., doi:10.1063/1.4816475.
- Li, Cheng, et al. “Manipulation of Nonlinear Optical Properties of Graphene Bonded Fiber Devices by Thermally Engineering Fermi-Dirac Distribution.” Advanced Optical Materials, vol. 5, no. 21, 2017, doi:10.1002/adom.201770103.
- Chirayath, V. A, et al. “Positron induced electron emission from graphene.” IOP Science, Journal of Physics: Conference Series.
- Lock, Evgeniya H., et al. Stable IR Transparent Conductive Graphene Hybrid Materials and Methods of Making.
- Beechem, Thomas E., et al. “Self-Heating and Failure in Scalable Graphene Devices.” Scientific Reports, vol. 6, no. 1, Sept. 2016, doi:10.1038/srep26457.
- Ma, Yufeng, and Anni Siitonen. Chemical sensor using molecularly-Imprinted single layer graphene.
- Morrow, Wayne K., et al. “Role of graphene interlayers in mitigating degradation of Ni/Au ohmic contact morphology on p-Type GaN.” Vacuum, vol. 128, 2016, pp. 34–38., doi:10.1016/j.vacuum.2016.03.004.
- Nagamanasa, Kandula Hima, et al. “Liquid-Cell Electron Microscopy of Adsorbed Polymers.” Advanced Materials, vol. 29, no. 41, 2017, p. 1703555., doi:10.1002/adma.201703555.
- Hui, Fei. “Variability of graphene devices fabricated using graphene inks: Atomic force microscope tips.” Surface and Coatings Technology, vol. 320, 25 June 2017, doi:10.1016/j.surfcoat.2016.12.020.
- Chirayath, V A, et al. “Investigation of graphene using low energy positron annihilation induced Doppler broadening spectroscopy.” Journal of Physics: Conference Series, vol. 791, 2017, p. 012032., doi:10.1088/1742-6596/791/1/012032.
- Niu, Tianxiao, et al. “Indentation behavior of the stiffest membrane mounted on a very compliant substrate: Graphene on PDMS.” International Journal of Solids and Structures, vol. 132-133, 2018, pp. 1–8., doi:10.1016/j.ijsolstr.2017.05.038.
- Nashed, Ramy, et al. “Ultra-High Mobility in Dielectrically Pinned CVD Graphene.” IEEE Journal of the Electron Devices Society, vol. 4, no. 6, 2016, pp. 466–472., doi:10.1109/jeds.2016.2595498.
- Niu, Tianxiao, et al. “Fracture behavior of graphene mounted on stretchable substrate.” Carbon, vol. 109, 2016, pp. 852–859., doi:10.1016/j.carbon.2016.08.087.
- Boutilier, Michael S H, et al. “Knudsen effusion through polymer-Coated three-Layer porous graphene membranes.” Nanotechnology, vol. 28, no. 18, Oct. 2017, p. 184003., doi:10.1088/1361-6528/aa680f.
- Du, Feng, et al. “Surface stress of graphene layers supported on soft substrate.” Scientific Reports, vol. 6, no. 1, Nov. 2016, doi:10.1038/srep25653.
- Yokaribas, Volkan, et al. “Strain Gauges Based on CVD Graphene Layers and Exfoliated Graphene Nanoplatelets with Enhanced Reproducibility and Scalability for Large Quantities.” Sensors, vol. 17, no. 12, 2017, p. 2937., doi:10.3390/s17122937.
- Kuru, Cihan, et al. “Enhanced Power Conversion Efficiency of Graphene/Silicon Heterojunction Solar Cells Through NiO Induced Doping.” Journal of Nanoscience and Nanotechnology, vol. 16, no. 1, Jan. 2016, pp. 1190–1193., doi:10.1166/jnn.2016.12079.
- Korzeniewski, Carol, Jay P. Kitt, Saheed Bukola, Stephen E. Creager, Shelley D. Minteer, and Joel M. Harris. "Single Layer Graphene for Estimation of Axial Spatial Resolution in Confocal Raman Microscopy Depth Profiling." Analytical chemistry 91, no. 1 (2018): 1049-1055.
- Mackin, Charles E., and Tomás Apostol Palacios. "Sensor systems and related fabrication techniques." U.S. Patent Application 15/773,247, filed November 8, 2018.
- Wu, Ting, Abdullah Alharbi, Takashi Taniguchi, Kenji Watanabe, and Davood Shahrjerdi. "Low-frequency noise in irradiated graphene FETs." Applied Physics Letters 113, no. 19 (2018): 193502.
- Xie, Yadian. "Study on high-purity alumina preparation and its applications." PhD diss., Université du Québec à Chicoutimi, 2017.
- Liu, Siyang, Myungji Kim, and Hong Koo Kim. "Electrically-triggered micro-explosion in a graphene/SiO 2/Si structure." Scientific reports 8, no. 1 (2018): 7379.
- Ulibarri, Agatha. "Measuring the Double Layer Capacitance of Electrolyte Solutions Using a Graphene Field Effect Transistor." (2018).
- Das, Sonali, Mohammad Jobayer Hossain, Siu-Fung Leung, Anya Lenox, Yeonwoong Jung, Kristopher Davis, Jr-Hau He, and Tania Roy. "A leaf-inspired photon management scheme using optically tuned bilayer nanoparticles for ultra-thin and highly efficient photovoltaic devices." Nano Energy 58 (2019): 47-56.
- Laua, K. Y., A. A. Latifb, MH Abu Bakara, and M. A. Mahdia. "HIGH SIGNAL-TO-NOISE RATIO Q-SWITCHING ERBIUM DOPED FIBER LASER PULSE EMISSION UTILIZING SINGLE LAYER TRIVIAL TRANSFER GRAPHENE FILM SATURABLE ABSORBER." JURNAL TEKNOLOGI 78, no. 3 (2016): 129-133.
- Carmichael, Christopher P., M. Scott Smith, Arthur R. Weeks, and Donald C. Malocha. "Experimental Investigation of Surface Acoustic Wave Acoustoelectric Effect using a Graphene Film on Lithium Niobate." IEEE transactions on ultrasonics, ferroelectrics, and frequency control 65, no. 11 (2018): 2205-2207.
- Fujimoto, Akira, Christopher J. Perini, Daiju Terasawa, Akira Fukuda, Yoshiyuki Harada, Shigehiko Sasa, Mitsuaki Yano, and Eric M. Vogel. "Disorder and Weak Localization near Charge Neutral Point in Ti‐cleaned Single‐Layer Graphene." physica status solidi (b) (2019): 1800541.
- Zhao, Jingyi, Zhengmin Cao, Yuqing Cheng, Jianning Xu, Te Wen, Aiqin Hu, Qihuang Gong, and Guowei Lu. "In situ Optical Study of Gold Nanorod Coupling with Graphene." Advanced Optical Materials 6, no. 8 (2018): 1701043.
- Su, Fang, Zhaohao Zhang, Shasha Li, Peian Li, and Tao Deng. "Long-term stability of photodetectors based on graphene field-effect transistors encapsulated with Si3N4 layers." Applied Surface Science 459 (2018): 164-170.
- Ulibarri, Agatha. "Measuring the Double Layer Capacitance of Electrolyte Solutions Using a Graphene Field Effect Transistor." (2018).
- Dong, Nannan, Sumei Wang, Lan Jiang, Yi Jiang, Peng Wang, and Liuchao Zhang. "Pressure and temperature sensor based on graphene diaphragm and fiber Bragg gratings." IEEE Photonics Technology Letters 30, no. 5 (2018): 431-434.
- Lau, K. Y., MH Abu Bakar, AR Md Zain, A. F. Abas, M. T. Alresheedi, and M. A. Mahdi. "Stable multi-wavelength erbium-doped fiber laser assisted by graphene/PMMA thin film." Optics & Laser Technology 105 (2018): 129-134.
- Herekar, Satish. "Systems, methods, and kits to reduce surface heating during tissue treatment." U.S. Patent Application 15/896,886, filed June 28, 2018.
- Xu, Wendao, Lijuan Xie, Jianfei Zhu, Longhua Tang, Ranjan Singh, Chen Wang, Yungui Ma, Hou-Tong Chen, and Yibin Ying. "Terahertz biosensing with a graphene-metamaterial heterostructure platform." Carbon 141 (2019): 247-252.
- Deng, Tao, Zhaohao Zhang, Yaxuan Liu, Yingxin Wang, Fang Su, Shasha Li, Yang Zhang et al. "Three-dimensional graphene field-effect transistors as high-performance photodetectors." Nano letters (2019).
- Barnard, H. R., E. Zossimova, N. H. Mahlmeister, L. M. Lawton, I. J. Luxmoore, and G. R. Nash. "Boron nitride encapsulated graphene infrared emitters." Applied Physics Letters 108, no. 13 (2016): 131110.
- Wu, Xiangyu, Inge Asselberghs, Cedric Huyghebaert, Antonino Contino, Bart Soree, Marc Heyns, and Zsolt Tokei. "Graphene Interconnects-High Performance Twisted 20 nm Graphene Ribbons." In 2018 IEEE International Interconnect Technology Conference (IITC), pp. 25-27. IEEE, 2018.
- Li, Cheng, Xiyu Yu, Tian Lan, Jian Liu, and Shangchun Fan. "Insensitivity to Humidity in Fabry–Perot Sensor With Multilayer Graphene Diaphragm." IEEE Photonics Technology Letters 30, no. 6 (2018): 565-568.
- Dong, Nannan, and Bo Yang. "Simultaneous Measurement of Pressure and Temperature Based on Graphene." In IOP Conference Series: Materials Science and Engineering, vol. 382, no. 5, p. 052011. IOP Publishing, 2018.
- Zhang, Yingjie, Youngseok Kim, Matthew J. Gilbert, and Nadya Mason. "Electronic transport in a two-dimensional superlattice engineered via self-assembled nanostructures." npj 2D Materials and Applications 2, no. 1 (2018): 31.
- Mackin, Charles Edward. "Graphene chemical and biological sensors: modeling, systems, and applications." PhD diss., Massachusetts Institute of Technology, 2018.
- Yin, Yin, Jinbo Pang, Jiawei Wang, Xueyi Lu, Qi Hao, Ehsan Saei Ghareh Naz, Xinxing Zhou, Libo Ma, and Oliver G. Schmidt. "Graphene-Activated Optoplasmonic Nanomembrane Cavities for Photodegradation Detection." ACS applied materials & interfaces (2019).
- Andoy, Nesha M., Marcin S. Filipiak, Daniel Vetter, Óscar Gutiérrez‐Sanz, and Alexey Tarasov. "Graphene‐Based Electronic Immunosensor with Femtomolar Detection Limit in Whole Serum." Advanced Materials Technologies 3, no. 12 (2018): 1800186.
- Schweizer, Peter, Christian Dolle, and Erdmann Spiecker. "In situ manipulation and switching of dislocations in bilayer graphene." Science advances 4, no. 8 (2018): eaat4712.
- Hutzler, Andreas, Christian David Matthus, Christian Dolle, Mathias Rommel, Michael PM Jank, Erdmann Spiecker, and Lothar Frey. "Large-Area Layer Counting of Two-Dimensional Materials Evaluating the Wavelength Shift in Visible-Reflectance Spectroscopy." The Journal of Physical Chemistry C (2019).
- Hu, Ming, Yucong Yan, Kun Huang, Afzal Khan, Xiaodong Qiu, Dikai Xu, Hui Zhang, Xuegong Yu, and Deren Yang. "Performance Improvement of Graphene/Silicon Photodetectors Using High Work Function Metal Nanoparticles with Plasma Effect." Advanced Optical Materials 6, no. 9 (2018): 1701243.
- Hu, Shu-Kai, Fang-Yen Lo, Chih-Chen Hsieh, and Ling Chao. "Sensing Ability and Formation Criterion of Fluid Supported Lipid Bilayer Coated Graphene Field-Effect Transistors." ACS sensors (2019).
- Nemani, Srinivasa Kartik, Dayong Chen, Marwan H. Mohamed, and Hossein Sojoudi. "Stretchable and hydrophobic electrochromic devices using wrinkled graphene and PEDOT: PSS." Journal of Nanomaterials 2018 (2018).
- Wang, Kenan. "The Incorporation of Graphene to Lithium Cobalt Oxide as a Cathode to Improve the Performance of Lithium Ion Batteries." (2018).
- Cho, Hoduk. "The Use of Liquid Phase Transmission Electron Microscopy for Quantifying Interactions Between Colloidal Nanoparticles and Visualizing Their Self-Assembled Structures." PhD diss., UC Berkeley, 2018.
- Goldflam, Michael D., Isaac Ruiz, Stephen W. Howell, Joel R. Wendt, Michael B. Sinclair, David W. Peters, and Thomas E. Beechem. "Tunable dual-band graphene-based infrared reflectance filter." Optics express 26, no. 7 (2018): 8532-8541.
- Hutzler, A., C. D. Matthus, M. Rommel, and L. Frey. "Generalized approach to design multi-layer stacks for enhanced optical detectability of ultrathin layers." Applied Physics Letters 110, no. 2 (2017): 021909.
|
-
FAQ
1. What's the difference between CVD graphene on copper foil and CVD graphene on copper foil (graphene factory)?
Our CVD Graphene on Copper Foil (Graphene Factory) product is designed for large size wafer transfers (up to 6x6in) and currently only the monolayer type is available. As the size increases there are likely to be more defects as compared to our standard CVD Graphene on Cu Foil. In general, our standard CVD Graphene on Copper Foil has better quality and the size is available up to 6inch x 4inch. This one has better monolayer graphene coverage because it is easier to control for smaller sizes. Both products are prepared by the CVD method, but we use a different set of equipment, so we name the products differently to make that distinction. You may choose whichever works best for your application.
|
-
Q&A
1. What is the thickness of PMMA?
About 500nm.
Research Citations of ACS Material Products
- Mackin, Charles, and Tomás Palacios. “Large-Scale sensor systems based on graphene electrolyte-Gated field-Effect transistors.” The Analyst, vol. 141, no. 9, 2016, pp. 2704–2711., doi:10.1039/c5an02328a.
- Bajestani, Raeis Zadeh, and Seyyed Mohsen. “Graphene-Based Nanophotonic Structures.” University of Waterloo, 2016.
- Nieto, Andy, et al. “Graphene reinforced metal and ceramic matrix composites: a review.” International Materials Reviews, vol. 62, no. 5, 27 Oct. 2016, doi:10.1080/09506608.2016.1219481.
- Hutzler, A., et al. “Generalized approach to design multi-Layer stacks for enhanced optical detectability of ultrathin layers.” Applied Physics Letters, vol. 110, no. 2, Sept. 2017, p. 021909., doi:10.1063/1.4973968.
|
-
FAQ
1. Is the CVD Graphene size the same as the PET Substrate size?
Our CVD Graphene on PET Substrate product has handling space, making the PET substrate a larger size than the CVD graphene size. This handling space enables the user to handle the PET substrate without damaging the CVD Graphene portion. This handling space of 0.50cm applies to all the standard sizes we offer it in.
Research Citations of ACS Material Products
- Chen, Ruiyi, et al. “Co-Percolating Graphene-Wrapped Silver Nanowire Network for High Performance, Highly Stable, Transparent Conducting Electrodes.” Advanced Functional Materials, vol. 23, no. 41, 2013, pp. 5150–5158., doi:10.1002/adfm.201300124.
- O’Hern, Sean C., et al. “Selective Molecular Transport through Intrinsic Defects in a Single Layer of CVD Graphene.” ACS Nano, vol. 6, no. 11, Sept. 2012, pp. 10130–10138., doi:10.1021/nn303869m.
- Yoo, Jae-Hyuck, et al. “Graphene folds by femtosecond laser ablation.” Applied Physics Letters, vol. 100, no. 23, Apr. 2012, p. 233124., doi:10.1063/1.4724213.
- Longchamp, Jean-Nicolas, et al. “Low-Energy electron transmission imaging of clusters on free-Standing graphene.” Applied Physics Letters, vol. 101, no. 11, Oct. 2012, p. 113117., doi:10.1063/1.4752717.
- Chen, Xu-Dong, et al. “High-Quality and efficient transfer of large-Area graphene films onto different substrates.” Carbon, vol. 56, May 2013, pp. 271–278., doi:10.1016/j.carbon.2013.01.011.
- Ye, Qing., et al. “Polarization-Dependent optical absorption of graphene under total internal reflection.” Applied Physics Letters, vol. 102, no. 2, doi:10.1063/1.4776694.
- Longchamp, Jean-Nicolas, et al. “Ultraclean freestanding graphene by platinum-Metal catalysis.” Journal of Vacuum Science & Technology B, Nanotechnology and Microelectronics: Materials, Processing, Measurement, and Phenomena, vol. 31, no. 2, 2013, p. 020605., doi:10.1116/1.4793746.
- Wang, Yung Yu, and Peter J. Burke. “A large-Area and contamination-Free graphene transistor for liquid-Gated sensing applications.” Applied Physics Letters, vol. 103, no. 5, 2013, p. 052103., doi:10.1063/1.4816764.
- Wang, Peng, et al. “Accurate layers determination of graphene on transparent substrate based on polarization-Sensitive absorption effect.” Applied Physics Letters, vol. 103, no. 18, 2013, p. 181902., doi:10.1063/1.4827812.
- Zhou, Rong, et al. “Large-Energy, narrow-Bandwidth laser pulse at 1645 nm in a diode-Pumped Er:YAG solid-State laser passively Q-Switched by a monolayer graphene saturable absorber.” Applied Optics, vol. 53, no. 2, Sept. 2014, p. 254., doi:10.1364/ao.53.000254.
- Choi, Duyoung, et al. “Nanopatterned Graphene Field Effect Transistor Fabricated Using Block Co-Polymer Lithography.” Materials Research Letters, vol. 2, no. 3, Sept. 2014, pp. 131–139., doi:10.1080/21663831.2013.876676.
- Srisonphan, Siwapon, et al. “Space charge neutralization by electron-Transparent suspended graphene.” Scientific Reports, vol. 4, no. 1, 2014, doi:10.1038/srep03764.
- Joiner, C. A., et al. “Cleaning graphene with a titanium sacrificial layer.” Applied Physics Letters, vol. 104, no. 22, Feb. 2014, p. 223109., doi:10.1063/1.4881886.
- Roy, T., et al. “Tunneling characteristics in chemical vapor deposited graphene–hexagonal boron nitride–graphene junctions.” Applied Physics Letters, vol. 104, no. 12, 2014, p. 123506., doi:10.1063/1.4870073.
- Burke, Peter J. “Charging the Quantum Capacitance of Graphene with a Single Biological Ion Channel.” Biophysical Journal, vol. 106, no. 2, 2014, doi:10.1016/j.bpj.2013.11.2796.
- Li, Peining, et al. “Graphene-Enhanced Infrared Near-Field Microscopy.” Nano Letters, vol. 14, no. 8, 2014, pp. 4400–4405., doi:10.1021/nl501376a.
- Boutilier, Michael S. H., et al. “Implications of Permeation through Intrinsic Defects in Graphene on the Design of Defect-Tolerant Membranes for Gas Separation.” ACS Nano, vol. 8, no. 1, Mar. 2014, pp. 841–849., doi:10.1021/nn405537u.
- Lee, Jiye, et al. “Switching Individual Quantum Dot Emission through Electrically Controlling Resonant Energy Transfer to Graphene.” Nano Letters, vol. 14, no. 12, 2014, pp. 7115–7119., doi:10.1021/nl503587z.
- Srisonphan, Siwapon, and Komsan Hongesombut. “Tuning the ballistic electron transport of spatial graphene–metal sandwich electrode on a vacuum-Silicon-Based device.” RSC Advances, vol. 5, no. 3, 2015, pp. 2032–2037., doi:10.1039/c4ra09503k.
- Hui, Fei, et al. “Mechanical properties of locally oxidized graphene electrodes.” Archive of Applied Mechanics, vol. 85, no. 3, 2014, pp. 339–345., doi:10.1007/s00419-014-0957-4.
- Fujimoto, A, et al. “Negative magnetoresistance in Ti-Cleaned single-Layer graphene.” Journal of Physics: Conference Series, vol. 603, 2015, p. 012021., doi:10.1088/1742-6596/603/1/012021.
- Thareja, Vrinda, et al. “Electrically Tunable Coherent Optical Absorption in Graphene with Ion Gel.” Nano Letters, vol. 15, no. 3, 2015, pp. 1570–1576., doi:10.1021/nl503431d.
- Ye, X. H., et al. “Corrosion resistance of graphene directly and locally grown on bulk nickel substrate by laser irradiation.” RSC Advances, vol. 5, no. 45, 2015, pp. 35384–35390., doi:10.1039/c5ra01267h.
- Horvath, Cameron . “Fabrication and Characterization of Edge-Conformed Graphene-Silicon Waveguides.” IEEE Photonics Technology Letters, vol. 27, no. 6, pp. 585–587., doi:10.1109/LPT.2014.2385757.
- Marta, Bogdan, et al. “Efficient etching-Free transfer of high quality, large-Area CVD grown graphene onto polyvinyl alcohol films.” Applied Surface Science, vol. 363, 2016, pp. 613–618., doi:10.1016/j.apsusc.2015.11.265.
- Zheng, Guanpeng, et al. “Improved Transfer Quality of CVD-Grown Graphene by Ultrasonic Processing of Target Substrates: Applications for Ultra-Fast Laser Photonics.” ACS Applied Materials & Interfaces, vol. 5, no. 20, Sept. 2013, pp. 10288–10293., doi:10.1021/am403205v.
- Zeng, Yong, et al. “Investigate the interface structure and growth mechanism of high quality ZnO films grown on multilayer graphene layers.” Applied Surface Science, vol. 301, 2014, pp. 391–395., doi:10.1016/j.apsusc.2014.02.088.
- Ye, Qing, et al. “Polarization-Dependent optical absorption of graphene under total internal reflection.” Applied Physics Letters, vol. 102, no. 2, Jan. 2013, doi:10.1063/1.4776694.
- Watanabe, Hiroshi, et al. “Layer number dependence of carrier lifetime in graphenes observed using time-Resolved mid-Infrared luminescence.” Chemical Physics Letters, vol. 637, 2015, pp. 58–62., doi:10.1016/j.cplett.2015.07.046.
- He, Yingbo, et al. “Strongly enhanced Raman scattering of graphene by a single gold nanorod.” Applied Physics Letters, vol. 107, no. 5, Mar. 2015, p. 053104., doi:10.1063/1.4927759.
- Choi, Duyoung, et al. “Uniformly Nanopatterned Graphene Field-Effect Transistors with Enhanced Properties.” Nanoscale Research Letters, vol. 10, no. 1, Nov. 2015, doi:10.1186/s11671-015-0976-2.
- Miao, Lili, et al. “Broadband ultrafast nonlinear optical response of few-Layers graphene: toward the mid-Infrared regime.” Photonics Research, vol. 3, no. 5, 2015, p. 214., doi:10.1364/prj.3.000214.
- Hu, Jianchen, et al. “Improvement of the electrical contact resistance at rough interfaces using two dimensional materials.” Journal of Applied Physics, vol. 118, no. 21, July 2015, p. 215301., doi:10.1063/1.4936366.
- Jain, Tarun, et al. “Heterogeneous sub-Continuum ionic transport in statistically isolated graphene nanopores.” Nature Nanotechnology, vol. 10, no. 12, May 2015, pp. 1053–1057., doi:10.1038/nnano.2015.222.
- Srisonphan, Siwapon, and Komsan Hongesombut. “Tuning the ballistic electron transport of spatial graphene–metal sandwich electrode on a vacuum-Silicon-Based device.” RSC Advances, vol. 5, no. 3, 2015, pp. 2032–2037., doi:10.1039/c4ra09503k.
- Liu, Xiangjiang, et al. “Compact Shielding of Graphene Monolayer Leads to Extraordinary SERS-Active Substrate with Large-Area Uniformity and Long-Term Stability.” Scientific Reports, vol. 5, no. 1, 2015, doi:10.1038/srep17167.
- Hui, Fei, et al. “Moving graphene devices from lab to market: advanced graphene-Coated nanoprobes.” Nanoscale, vol. 8, no. 16, 2016, pp. 8466–8473., doi:10.1039/c5nr06235g.
- Ye, Xiaohui, et al. “Protecting carbon steel from corrosion by laser in situ grown graphene films.” Carbon, vol. 94, 2015, pp. 326–334., doi:10.1016/j.carbon.2015.06.080.
- Wu, Man, et al. “Wavelength switchable graphene Q-Switched fiber laser with cascaded fiber Bragg gratings.” Optics Communications, vol. 368, 2016, pp. 81–85., doi:10.1016/j.optcom.2016.01.069.
- Mackin, Charles, and Tomás Palacios. “Large-Scale sensor systems based on graphene electrolyte-Gated field-Effect transistors.” The Analyst, vol. 141, no. 9, 2016, pp. 2704–2711., doi:10.1039/c5an02328a.
- Politou, Maria, et al. “Multi-Layer graphene interconnect.” 2016 IEEE International Interconnect Technology Conference / Advanced Metallization Conference (IITC/AMC), July 2016, doi:10.1109/IITC-AMC.2016.7507731.
- Cao, Zhengmin, et al. “Nano-Gap between a gold tip and nanorod for polarization dependent surface enhanced Raman scattering.” Applied Physics Letters, vol. 109, no. 23, May 2016, p. 233103., doi:10.1063/1.4971832.
- Deng, Xiangquan, et al. “Terahertz-Induced photothermoelectric response in graphene-Metal contact structures.” Journal of Physics D: Applied Physics, vol. 49, no. 42, 2016, p. 425101., doi:10.1088/0022-3727/49/42/425101.
- Guo, Chu-Cai, et al. “Experimental Demonstration of Total Absorption over 99% in the Near Infrared for Monolayer-Graphene-Based Subwavelength Structures.” Advanced Optical Materials, vol. 4, no. 12, Jan. 2016, pp. 1955–1960., doi:10.1002/adom.201600481.
- Hu, Jianbo, et al. “Rippling ultrafast dynamics of suspended 2D monolayers, graphene.” Proceedings of the National Academy of Sciences, vol. 113, no. 43, Oct. 2016, doi:10.1073/pnas.1613818113.
- Li, Cheng, et al. “Measurement of the Adhesion Energy of Pressurized Graphene Diaphragm Using Optical Fiber Fabry–Perot Interference.” IEEE Sensors Journal, vol. 16, no. 10, 2016, pp. 3664–3669., doi:10.1109/jsen.2016.2536783.
- Yuan, Jun, et al. “Modulation of far-Infrared light transmission by graphene-Silicon Schottky junction.” Optical Materials Express, vol. 6, no. 12, 2016, p. 3908., doi:10.1364/ome.6.003908.
- Li, Cheng, et al. “Measurement of thermal expansion coefficient of graphene diaphragm using optical fiber Fabry–Perot interference.” Measurement Science and Technology, vol. 27, no. 7, 2016, p. 075102., doi:10.1088/0957-0233/27/7/075102.
- Kafiah, Feras M., et al. “Monolayer graphene transfer onto polypropylene and polyvinylidenedifluoride microfiltration membranes for water desalination.” Desalination, vol. 388, 2016, pp. 29–37., doi:10.1016/j.desal.2016.02.027.
- Wu, Xiangyu, et al. “Doping of graphene for the application in nano-Interconnect.” Microelectronic Engineering, vol. 167, 5 Jan. 2017, pp. 42–46., doi:10.1016/j.mee.2016.10.013.
- Liu, Siyang, et al. “Atomic emission spectroscopy of electrically triggered exploding nanoparticle analytes on graphene/SiO2/Si substrate.” Journal of Vacuum Science & Technology B, Nanotechnology and Microelectronics: Materials, Processing, Measurement, and Phenomena, vol. 34, no. 6, 2016, doi:10.1116/1.4964819.
- Sattler, Klaus D. Carbon nanomaterials sourcebook. Vol. 1, CRC Press, 2016.
- Politou, Maria, et al. “Evaluation of multilayer graphene for advanced interconnects.” Microelectronic Engineering, vol. 167, 5 Jan. 2017, pp. 1–5., doi:10.1016/j.mee.2016.09.011.
- Hwang, Michael T., et al. “Highly specific SNP detection using 2D graphene electronics and DNA strand displacement.” Proceedings of the National Academy of Sciences, vol. 113, no. 26, 2016, pp. 7088–7093., doi:10.1073/pnas.1603753113.
- Nieto, Andy. “Graphene reinforced metal and ceramic matrix composites: a review.” International Materials Reviews, vol. 62, no. 5, 27 Oct. 2017, pp. 241–302., doi:10.1080/09506608.2016.1219481.
- Khorasaninejad, M., et al. “Highly Enhanced Raman Scattering of Graphene using Plasmonic Nano-Structure.” Scientific Reports, vol. 3, no. 1, 2013, doi:10.1038/srep02936.
- Kunz, Daniel A., et al. “Space-Resolved In-Plane Moduli of Graphene Oxide and Chemically Derived Graphene Applying a Simple Wrinkling Procedure (Adv. Mater. 9/2013).” Advanced Materials, vol. 25, no. 9, Apr. 2013, pp. 1336–1336., doi:10.1002/adma.201370060.
- Peng, Shuhua, et al. “Microwetting of Supported Graphene on Hydrophobic Surfaces Revealed by Polymerized Interfacial Femtodroplets.” Langmuir, vol. 30, no. 33, 2014, pp. 10043–10049., doi:10.1021/la5022774.
- Luo, Wen, et al. “Single-Layer Graphene as an Effective Mediator of the Metal–Support Interaction.” The Journal of Physical Chemistry Letters, vol. 5, no. 11, 2014, pp. 1837–1844., doi:10.1021/jz500425j.
- Suemoto, Tohru, et al. “Thickness dependent hot-Phonon effects observed by femtosecond mid-Infrared luminescence in graphene.” 19th International Conference on Ultrafast Phenomena, 2014, doi:10.1364/up.2014.07.mon.p1.32.
- Zhou, Haosen, et al. “Influence of CO2 On the Stability of Discharge Performance for Li-Air Battery with Hybrid Electrolyte Based On the Graphene Sheet.” Conference: 224th ECS Meeting. Oct. 2013, doi:10.1039/c3ra47258b.
- Thareja, Vrinda. “Electrically Tunable Optical Absorption in a Graphene-Based Salisbury Screen.” CORNELL UNIVERSITY LIBRARY, 7 Sept. 2014.
- Horvath, Cameron S. “Light Propagation and Photothermal Nonlinearity in Graphene-Si Waveguides .” University of Alberta Libraries , Nov. 2013, doi:10.7939/R3ST1N.
- Li, Cheng, et al. “Interference characteristics in a Fabry–Perot cavity with graphene membrane for optical fiber pressure sensors.” Microsystem Technologies, vol. 21, no. 11, 2014, pp. 2297–2306., doi:10.1007/s00542-014-2333-2.
- Kou, Jun-Long, et al. “Platform for enhanced light–graphene interaction length and miniaturizing fiber stereo devices.” Optica, vol. 1, no. 5, 2014, p. 307., doi:10.1364/optica.1.000307.
- Karnik, Rohit. “Ionic and Molecular Transport Through Graphene Membranes.” Transport and Reactivity of Solutions in Confined Hydrosystems NATO Science for Peace and Security Series C: Environmental Security, Dec. 2013, pp. 95–102., doi:10.1007/978-94-007-7534-3_8.
- Warren, A. J., et al. “In vacuo X-Ray data collection from graphene-Wrapped protein crystals.” Acta Crystallographica Section D STRUCTURAL BIOLOGY, vol. D71, 2015, pp. 2079–2088., doi:10.1107/S1399004715014194.
- Wen, Chenyu, et al. “Assessing kinetics of surface adsorption–desorption of gas molecules via electrical measurements.” Sensors and Actuators B: Chemical, vol. 223, 2016, pp. 791–798., doi:10.1016/j.snb.2015.10.019.
- Li, Cheng, et al. “Analyzing the applicability of miniature ultra-High sensitivity Fabry–Perot acoustic sensor using a nanothick graphene diaphragm.” Measurement Science and Technology, vol. 26, no. 8, 10 July 2015.
- Li, Cheng, et al. “Analyzing the temperature sensitivity of Fabry-Perot sensor using multilayer graphene diaphragm.” OSA Publishing, vol. 23, no. 21, 2015, pp. 27494–27502., doi:10.1364/OE.23.027494.
- Lau, K. Y., et al. “High Signal-To-Noise Ratio Q-Switching Erbium Doped Fiber Laser Pulse Emission Utilizing Single Layer Trivial Transfer Graphene Film Saturable Absorber.” Jurnal Teknologi, vol. 78, no. 3, 2016, doi:10.11113/jt.v78.7478.
- Zheng, Shijun, et al. “Acoustic charge transport induced by the surface acoustic wave in chemical doped graphene.” Applied Physics Letters, vol. 109, no. 18, 2016, p. 183110., doi:10.1063/1.4967192.
- Deng, Xiangquan, et al. “Terahertz-Induced photothermoelectric response in graphene-Metal contact structures.” Journal of Physics D: Applied Physics, vol. 49, no. 42, 2016, p. 425101., doi:10.1088/0022-3727/49/42/425101.
- Luo, Wen, and Spyridon Zafeiratos. “Inside Back Cover: Graphene-Coated ZnO and SiO2 as Supports for CoO Nanoparticles with Enhanced Reducibility.” ChemPhysChem, vol. 17, no. 19, Apr. 2016, pp. 3146–3146., doi:10.1002/cphc.201601023.
- Lau, K.Y., et al. “Passively mode-Locked soliton femtosecond pulses employing graphene saturable absorber.” Optics & Laser Technology, vol. 94, 2017, pp. 221–227., doi:10.1016/j.optlastec.2017.03.035.
- Fitri, Meika Aidil, et al. “Fabrication of TiO 2 -Graphene photocatalyst by direct chemical vapor deposition and its anti-Fouling property.” Materials Chemistry and Physics, vol. 198, 2017, pp. 42–48., doi:10.1016/j.matchemphys.2017.05.053.
- Li, Cheng, et al. “Nondestructive andin situdetermination of graphene layers using optical fiber Fabry–Perot interference.” Measurement Science and Technology, vol. 28, no. 2, Dec. 2017, p. 025206., doi:10.1088/1361-6501/aa54f8.
- Gao, Xiangyang, et al. “Measuring Graphene Adhesion on Silicon Substrate by Single and Dual Nanoparticle-Loaded Blister.” Advanced Materials Interfaces, vol. 4, no. 9, 2017, p. 1601023., doi:10.1002/admi.201601023.
- Tang, Xiaoduan, et al. “Five Orders of Magnitude Reduction in Energy Coupling across Corrugated Graphene/Substrate Interfaces.” ACS Applied Materials & Interfaces, vol. 6, no. 4, July 2014, pp. 2809–2818., doi:10.1021/am405388a.
- Morrow, W. K., et al. “(Invited) The Use of Graphene as a Solid State Diffusion Barrier.” ECS Transactions, vol. 61, no. 4, 2014, pp. 371–379., doi:10.1149/06104.0371ecst.
- Zheng, Haisheng, et al. “Ultrafine Pt nanoparticle induced doping/Strain of single layer graphene: experimental corroboration between conduction and Raman characteristics.” Journal of Materials Science: Materials in Electronics, vol. 26, no. 7, 2015, pp. 4746–4753., doi:10.1007/s10854-015-3043-y.
- Blinco , James P, et al. “Spin-Coated Carbon." Chemical Science, no. 9, 2013, pp. 3411–3415., doi:10.1039/C3SC51396C.
- Zheng, H., et al. “Effect of Sub 1-Nm Pt Nanoparticle on the Conduction Properties of Graphene Based Field Effect Transistor.” ECS Transactions, vol. 61, no. 39, Jan. 2014, pp. 1–11., doi:10.1149/06139.0001ecst.
- Mckitterick, Christopher B., et al. “Electron-Phonon cooling in large monolayer graphene devices.” Physical Review B, vol. 93, no. 7, Apr. 2016, doi:10.1103/physrevb.93.075410.
- Chan, Chun Yu. “Graphene based electrical biosensors for the detection of biomolecules.” The Hong Kong Polytechnic University, 2016.
- Pápa, Z., et al. “Spectroscopic ellipsometric investigation of graphene and thin carbon films from the point of view of depolarization effects.” Applied Surface Science, vol. 421, 2017, pp. 714–721., doi:10.1016/j.apsusc.2016.11.231.
- Hussain, Mushtaque, et al. “The improved piezoelectric properties of ZnO nanorods with oxygen plasma treatment on the single layer graphene coated polymer substrate.” Physica status solidi (a), vol. 211, no. 2, Oct. 2014, pp. 455–459., doi:10.1002/pssa.201300330.
- Kafiah, Feras, et al. “Synthesis of Graphene Based Membranes: Effect of Substrate Surface Properties on Monolayer Graphene Transfer.” Materials, vol. 10, no. 1, 2017, p. 86., doi:10.3390/ma10010086.
- Liang, Ji, et al. “Modulation of acousto-Electric current using a hybrid on-Chip AlN SAW/GFET device.” Applied Physics Letters, vol. 110, no. 24, Dec. 2017, p. 243504., doi:10.1063/1.4986481.
- Zhang, Yingjie & Kim, Youngseok & J. Gilbert, Matthew & Mason, Nadya. (2017). Electron transport in strain superlattices of graphene.
- Yan, Xiao-Qing, et al. “Polarization dependence of graphene transient optical response: interplay between incident direction and anisotropic distribution of nonequilibrium carriers.” Journal of the Optical Society of America B, vol. 34, no. 1, 2016, p. 218., doi:10.1364/josab.34.000218.
- Huang, Kun, et al. “Graphene coupled with Pt cubic nanoparticles for high performance, air-Stable graphene-Silicon solar cells.” Nano Energy, vol. 32, 2017, pp. 225–231., doi:10.1016/j.nanoen.2016.12.042.
- Lu, Bingyu, et al. “Roughened cylindrical gold layer with curve graphene coating for enhanced sensitivity of fiber SPR sensor.” 2017 19th International Conference on Solid-State Sensors, Actuators and Microsystems (TRANSDUCERS), 2017, doi:10.1109/transducers.2017.7994461.
- Kafiah, Feras, et al. “Synthesis of Graphene Based Membranes: Effect of Substrate Surface Properties on Monolayer Graphene Transfer.” Materials, vol. 10, no. 1, 2017, p. 86., doi:10.3390/ma10010086.
- Hsu, Wei-Hao, et al. “Low-Energy electron point projection microscopy/Diffraction study of suspended graphene.” Applied Surface Science, vol. 423, 30 Nov. 2017, pp. 266–274., doi:10.1016/j.apsusc.2017.06.148.
- Peng, Shuhua, et al. “Microwetting of Supported Graphene on Hydrophobic Surfaces Revealed by Polymerized Interfacial Femtodroplets.” Langmuir, vol. 30, no. 33, 2014, pp. 10043–10049., doi:10.1021/la5022774.
- Horvath, Cameron, et al. “Edge-Conformed silicon-Graphene waveguides: Fabrication and measurements.” 11th International Conference on Group IV Photonics (GFP), 2014, doi:10.1109/group4.2014.6961953.
- Watanabe, Hiroshi, et al. “Femtosecond midi-Infrared luminescence with hot-Phonon effects in graphenes and graphite.”
- Hassan, Saad. Transparent conductive article.
- Liang, Ji, et al. “Manipulation of carriers in graphene using an on-Chip acoustic wave device.” 2017 IEEE International Ultrasonics Symposium (IUS), 2017, doi:10.1109/ultsym.2017.8092898.
- Luo, Wen, et al. “Interaction of bimetallic PtCo layers with bare and graphene-Covered ZnO(0001) supports.” Surface Science, vol. 669, 2018, pp. 64–70., doi:10.1016/j.susc.2017.11.001.
- Jiang, Wen-Shuai, et al. “Preparation of high-Quality graphene using triggered microwave reduction under an air atmosphere.” Journal of Materials Chemistry C, 2018, doi:10.1039/c7tc03957c.
- Dong, Nannan, et al. “Pressure and Temperature Sensor Based on Graphene Diaphragm and Fiber Bragg Gratings.” IEEE Photonics Technology Letters, 2017, pp. 1–1., doi:10.1109/lpt.2017.2786292.
- Huczko, Andrzej, et al. “Efficient one-Pot combustion synthesis of few-Layered graphene.” Physica status solidi (b), vol. 252, no. 11, 2015, pp. 2412–2417., doi:10.1002/pssb.201552233.
- Lu, Bingyu, et al. “Roughened cylindrical gold layer with curve graphene coating for enhanced sensitivity of fiber SPR sensor.” 2017 19th International Conference on Solid-State Sensors, Actuators and Microsystems (TRANSDUCERS), 2017, doi:10.1109/transducers.2017.7994461.
- Fitri, Meika Aidil, et al. “Fabrication of TiO 2 -Graphene photocatalyst by direct chemical vapor deposition and its anti-Fouling property.” Materials Chemistry and Physics, vol. 198, 2017, pp. 42–48., doi:10.1016/j.matchemphys.2017.05.053.
- Bezryadin, A, et al. “Large energy storage efficiency of the dielectric layer of graphene nanocapacitors.” Nanotechnology, vol. 28, no. 49, 2017, p. 495401., doi:10.1088/1361-6528/aa935c.
- Shi, Cheng, et al. “Metamaterial-Based graphene thermal emitter.” Nano Research, June 2017, doi:10.1007/s12274-017-1922-7.
- McCaffrey, Debra L., et al. “Mechanism of ion adsorption to aqueous interfaces: Graphene/Water vs. air/Water.” Proceedings of the National Academy of Sciences, vol. 114, no. 51, 2017, pp. 13369–13373., doi:10.1073/pnas.1702760114.
- Zhang, Qin, et al. “Negative differential resistance and hysteresis in graphene-Based organic light-Emitting devices.” Journal of Materials Chemistry C, The Royal Society of Chemistry, 1 Jan. 2018, pubs.rsc.org/en/Content/ArticleLanding/2017/TC/C7TC05148D#!
- Miskin, Marc Z., et al. “Graphene-Based bimorphs for micron-Sized, autonomous origami machines.” Proceedings of the National Academy of Sciences, vol. 115, no. 3, Feb. 2018, pp. 466–470., doi:10.1073/pnas.1712889115.
- Bukola, Saheed, et al. “Selective Proton/Deuteron Transport through Nafion|Graphene|Nafion Sandwich Structures at High Current Density.” Journal of the American Chemical Society, vol. 140, no. 5, 2018, pp. 1743–1752., doi:10.1021/jacs.7b10853.
- Brown, Morgan A., et al. “Graphene Biotransistor Interfaced with a Nitrifying Biofilm.” Environmental Science & Technology Letters, vol. 2, no. 4, Mar. 2015, pp. 118–122., doi:10.1021/acs.estlett.5b00025.
- Woo, Sung Oh, and Winfried Teizer. “The effect of electron induced hydrogenation of graphene on its electrical transport properties.” Applied Physics Letters, vol. 103, no. 4, 2013, p. 041603., doi:10.1063/1.4816475.
- Li, Cheng, et al. “Manipulation of Nonlinear Optical Properties of Graphene Bonded Fiber Devices by Thermally Engineering Fermi-Dirac Distribution.” Advanced Optical Materials, vol. 5, no. 21, 2017, doi:10.1002/adom.201770103.
- Chirayath, V. A, et al. “Positron induced electron emission from graphene.” IOP Science, Journal of Physics: Conference Series.
- Lock, Evgeniya H., et al. Stable IR Transparent Conductive Graphene Hybrid Materials and Methods of Making.
- Beechem, Thomas E., et al. “Self-Heating and Failure in Scalable Graphene Devices.” Scientific Reports, vol. 6, no. 1, Sept. 2016, doi:10.1038/srep26457.
- Ma, Yufeng, and Anni Siitonen. Chemical sensor using molecularly-Imprinted single layer graphene.
- Morrow, Wayne K., et al. “Role of graphene interlayers in mitigating degradation of Ni/Au ohmic contact morphology on p-Type GaN.” Vacuum, vol. 128, 2016, pp. 34–38., doi:10.1016/j.vacuum.2016.03.004.
- Nagamanasa, Kandula Hima, et al. “Liquid-Cell Electron Microscopy of Adsorbed Polymers.” Advanced Materials, vol. 29, no. 41, 2017, p. 1703555., doi:10.1002/adma.201703555.
- Hui, Fei. “Variability of graphene devices fabricated using graphene inks: Atomic force microscope tips.” Surface and Coatings Technology, vol. 320, 25 June 2017, doi:10.1016/j.surfcoat.2016.12.020.
- Chirayath, V A, et al. “Investigation of graphene using low energy positron annihilation induced Doppler broadening spectroscopy.” Journal of Physics: Conference Series, vol. 791, 2017, p. 012032., doi:10.1088/1742-6596/791/1/012032.
- Niu, Tianxiao, et al. “Indentation behavior of the stiffest membrane mounted on a very compliant substrate: Graphene on PDMS.” International Journal of Solids and Structures, vol. 132-133, 2018, pp. 1–8., doi:10.1016/j.ijsolstr.2017.05.038.
- Nashed, Ramy, et al. “Ultra-High Mobility in Dielectrically Pinned CVD Graphene.” IEEE Journal of the Electron Devices Society, vol. 4, no. 6, 2016, pp. 466–472., doi:10.1109/jeds.2016.2595498.
- Niu, Tianxiao, et al. “Fracture behavior of graphene mounted on stretchable substrate.” Carbon, vol. 109, 2016, pp. 852–859., doi:10.1016/j.carbon.2016.08.087.
- Boutilier, Michael S H, et al. “Knudsen effusion through polymer-Coated three-Layer porous graphene membranes.” Nanotechnology, vol. 28, no. 18, Oct. 2017, p. 184003., doi:10.1088/1361-6528/aa680f.
- Du, Feng, et al. “Surface stress of graphene layers supported on soft substrate.” Scientific Reports, vol. 6, no. 1, Nov. 2016, doi:10.1038/srep25653.
- Yokaribas, Volkan, et al. “Strain Gauges Based on CVD Graphene Layers and Exfoliated Graphene Nanoplatelets with Enhanced Reproducibility and Scalability for Large Quantities.” Sensors, vol. 17, no. 12, 2017, p. 2937., doi:10.3390/s17122937.
- Kuru, Cihan, et al. “Enhanced Power Conversion Efficiency of Graphene/Silicon Heterojunction Solar Cells Through NiO Induced Doping.” Journal of Nanoscience and Nanotechnology, vol. 16, no. 1, Jan. 2016, pp. 1190–1193., doi:10.1166/jnn.2016.12079.
|
-
Research Citations of ACS Material Products
- Chen, Ruiyi, et al. “Co-Percolating Graphene-Wrapped Silver Nanowire Network for High Performance, Highly Stable, Transparent Conducting Electrodes.” Advanced Functional Materials, vol. 23, no. 41, 2013, pp. 5150–5158., doi:10.1002/adfm.201300124.
- O’Hern, Sean C., et al. “Selective Molecular Transport through Intrinsic Defects in a Single Layer of CVD Graphene.” ACS Nano, vol. 6, no. 11, Sept. 2012, pp. 10130–10138., doi:10.1021/nn303869m.
- Yoo, Jae-Hyuck, et al. “Graphene folds by femtosecond laser ablation.” Applied Physics Letters, vol. 100, no. 23, Apr. 2012, p. 233124., doi:10.1063/1.4724213.
- Longchamp, Jean-Nicolas, et al. “Low-Energy electron transmission imaging of clusters on free-Standing graphene.” Applied Physics Letters, vol. 101, no. 11, Oct. 2012, p. 113117., doi:10.1063/1.4752717.
- Chen, Xu-Dong, et al. “High-Quality and efficient transfer of large-Area graphene films onto different substrates.” Carbon, vol. 56, May 2013, pp. 271–278., doi:10.1016/j.carbon.2013.01.011.
- Ye, Qing., et al. “Polarization-Dependent optical absorption of graphene under total internal reflection.” Applied Physics Letters, vol. 102, no. 2, doi:10.1063/1.4776694.
- Longchamp, Jean-Nicolas, et al. “Ultraclean freestanding graphene by platinum-Metal catalysis.” Journal of Vacuum Science & Technology B, Nanotechnology and Microelectronics: Materials, Processing, Measurement, and Phenomena, vol. 31, no. 2, 2013, p. 020605., doi:10.1116/1.4793746.
- Wang, Yung Yu, and Peter J. Burke. “A large-Area and contamination-Free graphene transistor for liquid-Gated sensing applications.” Applied Physics Letters, vol. 103, no. 5, 2013, p. 052103., doi:10.1063/1.4816764.
- Wang, Peng, et al. “Accurate layers determination of graphene on transparent substrate based on polarization-Sensitive absorption effect.” Applied Physics Letters, vol. 103, no. 18, 2013, p. 181902., doi:10.1063/1.4827812.
- Zhou, Rong, et al. “Large-Energy, narrow-Bandwidth laser pulse at 1645 nm in a diode-Pumped Er:YAG solid-State laser passively Q-Switched by a monolayer graphene saturable absorber.” Applied Optics, vol. 53, no. 2, Sept. 2014, p. 254., doi:10.1364/ao.53.000254.
- Choi, Duyoung, et al. “Nanopatterned Graphene Field Effect Transistor Fabricated Using Block Co-Polymer Lithography.” Materials Research Letters, vol. 2, no. 3, Sept. 2014, pp. 131–139., doi:10.1080/21663831.2013.876676.
- Srisonphan, Siwapon, et al. “Space charge neutralization by electron-Transparent suspended graphene.” Scientific Reports, vol. 4, no. 1, 2014, doi:10.1038/srep03764.
- Joiner, C. A., et al. “Cleaning graphene with a titanium sacrificial layer.” Applied Physics Letters, vol. 104, no. 22, Feb. 2014, p. 223109., doi:10.1063/1.4881886.
- Roy, T., et al. “Tunneling characteristics in chemical vapor deposited graphene–hexagonal boron nitride–graphene junctions.” Applied Physics Letters, vol. 104, no. 12, 2014, p. 123506., doi:10.1063/1.4870073.
- Burke, Peter J. “Charging the Quantum Capacitance of Graphene with a Single Biological Ion Channel.” Biophysical Journal, vol. 106, no. 2, 2014, doi:10.1016/j.bpj.2013.11.2796.
- Li, Peining, et al. “Graphene-Enhanced Infrared Near-Field Microscopy.” Nano Letters, vol. 14, no. 8, 2014, pp. 4400–4405., doi:10.1021/nl501376a.
- Boutilier, Michael S. H., et al. “Implications of Permeation through Intrinsic Defects in Graphene on the Design of Defect-Tolerant Membranes for Gas Separation.” ACS Nano, vol. 8, no. 1, Mar. 2014, pp. 841–849., doi:10.1021/nn405537u.
- Lee, Jiye, et al. “Switching Individual Quantum Dot Emission through Electrically Controlling Resonant Energy Transfer to Graphene.” Nano Letters, vol. 14, no. 12, 2014, pp. 7115–7119., doi:10.1021/nl503587z.
- Srisonphan, Siwapon, and Komsan Hongesombut. “Tuning the ballistic electron transport of spatial graphene–metal sandwich electrode on a vacuum-Silicon-Based device.” RSC Advances, vol. 5, no. 3, 2015, pp. 2032–2037., doi:10.1039/c4ra09503k.
- Hui, Fei, et al. “Mechanical properties of locally oxidized graphene electrodes.” Archive of Applied Mechanics, vol. 85, no. 3, 2014, pp. 339–345., doi:10.1007/s00419-014-0957-4.
- Fujimoto, A, et al. “Negative magnetoresistance in Ti-Cleaned single-Layer graphene.” Journal of Physics: Conference Series, vol. 603, 2015, p. 012021., doi:10.1088/1742-6596/603/1/012021.
- Thareja, Vrinda, et al. “Electrically Tunable Coherent Optical Absorption in Graphene with Ion Gel.” Nano Letters, vol. 15, no. 3, 2015, pp. 1570–1576., doi:10.1021/nl503431d.
- Ye, X. H., et al. “Corrosion resistance of graphene directly and locally grown on bulk nickel substrate by laser irradiation.” RSC Advances, vol. 5, no. 45, 2015, pp. 35384–35390., doi:10.1039/c5ra01267h.
- Horvath, Cameron . “Fabrication and Characterization of Edge-Conformed Graphene-Silicon Waveguides.” IEEE Photonics Technology Letters, vol. 27, no. 6, pp. 585–587., doi:10.1109/LPT.2014.2385757.
- Marta, Bogdan, et al. “Efficient etching-Free transfer of high quality, large-Area CVD grown graphene onto polyvinyl alcohol films.” Applied Surface Science, vol. 363, 2016, pp. 613–618., doi:10.1016/j.apsusc.2015.11.265.
- Zheng, Guanpeng, et al. “Improved Transfer Quality of CVD-Grown Graphene by Ultrasonic Processing of Target Substrates: Applications for Ultra-Fast Laser Photonics.” ACS Applied Materials & Interfaces, vol. 5, no. 20, Sept. 2013, pp. 10288–10293., doi:10.1021/am403205v.
- Zeng, Yong, et al. “Investigate the interface structure and growth mechanism of high quality ZnO films grown on multilayer graphene layers.” Applied Surface Science, vol. 301, 2014, pp. 391–395., doi:10.1016/j.apsusc.2014.02.088.
- Ye, Qing, et al. “Polarization-Dependent optical absorption of graphene under total internal reflection.” Applied Physics Letters, vol. 102, no. 2, Jan. 2013, doi:10.1063/1.4776694.
- Watanabe, Hiroshi, et al. “Layer number dependence of carrier lifetime in graphenes observed using time-Resolved mid-Infrared luminescence.” Chemical Physics Letters, vol. 637, 2015, pp. 58–62., doi:10.1016/j.cplett.2015.07.046.
- He, Yingbo, et al. “Strongly enhanced Raman scattering of graphene by a single gold nanorod.” Applied Physics Letters, vol. 107, no. 5, Mar. 2015, p. 053104., doi:10.1063/1.4927759.
- Choi, Duyoung, et al. “Uniformly Nanopatterned Graphene Field-Effect Transistors with Enhanced Properties.” Nanoscale Research Letters, vol. 10, no. 1, Nov. 2015, doi:10.1186/s11671-015-0976-2.
- Miao, Lili, et al. “Broadband ultrafast nonlinear optical response of few-Layers graphene: toward the mid-Infrared regime.” Photonics Research, vol. 3, no. 5, 2015, p. 214., doi:10.1364/prj.3.000214.
- Hu, Jianchen, et al. “Improvement of the electrical contact resistance at rough interfaces using two dimensional materials.” Journal of Applied Physics, vol. 118, no. 21, July 2015, p. 215301., doi:10.1063/1.4936366.
- Jain, Tarun, et al. “Heterogeneous sub-Continuum ionic transport in statistically isolated graphene nanopores.” Nature Nanotechnology, vol. 10, no. 12, May 2015, pp. 1053–1057., doi:10.1038/nnano.2015.222.
- Srisonphan, Siwapon, and Komsan Hongesombut. “Tuning the ballistic electron transport of spatial graphene–metal sandwich electrode on a vacuum-Silicon-Based device.” RSC Advances, vol. 5, no. 3, 2015, pp. 2032–2037., doi:10.1039/c4ra09503k.
- Liu, Xiangjiang, et al. “Compact Shielding of Graphene Monolayer Leads to Extraordinary SERS-Active Substrate with Large-Area Uniformity and Long-Term Stability.” Scientific Reports, vol. 5, no. 1, 2015, doi:10.1038/srep17167.
- Hui, Fei, et al. “Moving graphene devices from lab to market: advanced graphene-Coated nanoprobes.” Nanoscale, vol. 8, no. 16, 2016, pp. 8466–8473., doi:10.1039/c5nr06235g.
- Ye, Xiaohui, et al. “Protecting carbon steel from corrosion by laser in situ grown graphene films.” Carbon, vol. 94, 2015, pp. 326–334., doi:10.1016/j.carbon.2015.06.080.
- Wu, Man, et al. “Wavelength switchable graphene Q-Switched fiber laser with cascaded fiber Bragg gratings.” Optics Communications, vol. 368, 2016, pp. 81–85., doi:10.1016/j.optcom.2016.01.069.
- Mackin, Charles, and Tomás Palacios. “Large-Scale sensor systems based on graphene electrolyte-Gated field-Effect transistors.” The Analyst, vol. 141, no. 9, 2016, pp. 2704–2711., doi:10.1039/c5an02328a.
- Politou, Maria, et al. “Multi-Layer graphene interconnect.” 2016 IEEE International Interconnect Technology Conference / Advanced Metallization Conference (IITC/AMC), July 2016, doi:10.1109/IITC-AMC.2016.7507731.
- Cao, Zhengmin, et al. “Nano-Gap between a gold tip and nanorod for polarization dependent surface enhanced Raman scattering.” Applied Physics Letters, vol. 109, no. 23, May 2016, p. 233103., doi:10.1063/1.4971832.
- Deng, Xiangquan, et al. “Terahertz-Induced photothermoelectric response in graphene-Metal contact structures.” Journal of Physics D: Applied Physics, vol. 49, no. 42, 2016, p. 425101., doi:10.1088/0022-3727/49/42/425101.
- Guo, Chu-Cai, et al. “Experimental Demonstration of Total Absorption over 99% in the Near Infrared for Monolayer-Graphene-Based Subwavelength Structures.” Advanced Optical Materials, vol. 4, no. 12, Jan. 2016, pp. 1955–1960., doi:10.1002/adom.201600481.
- Hu, Jianbo, et al. “Rippling ultrafast dynamics of suspended 2D monolayers, graphene.” Proceedings of the National Academy of Sciences, vol. 113, no. 43, Oct. 2016, doi:10.1073/pnas.1613818113.
- Li, Cheng, et al. “Measurement of the Adhesion Energy of Pressurized Graphene Diaphragm Using Optical Fiber Fabry–Perot Interference.” IEEE Sensors Journal, vol. 16, no. 10, 2016, pp. 3664–3669., doi:10.1109/jsen.2016.2536783.
- Yuan, Jun, et al. “Modulation of far-Infrared light transmission by graphene-Silicon Schottky junction.” Optical Materials Express, vol. 6, no. 12, 2016, p. 3908., doi:10.1364/ome.6.003908.
- Li, Cheng, et al. “Measurement of thermal expansion coefficient of graphene diaphragm using optical fiber Fabry–Perot interference.” Measurement Science and Technology, vol. 27, no. 7, 2016, p. 075102., doi:10.1088/0957-0233/27/7/075102.
- Kafiah, Feras M., et al. “Monolayer graphene transfer onto polypropylene and polyvinylidenedifluoride microfiltration membranes for water desalination.” Desalination, vol. 388, 2016, pp. 29–37., doi:10.1016/j.desal.2016.02.027.
- Wu, Xiangyu, et al. “Doping of graphene for the application in nano-Interconnect.” Microelectronic Engineering, vol. 167, 5 Jan. 2017, pp. 42–46., doi:10.1016/j.mee.2016.10.013.
- Liu, Siyang, et al. “Atomic emission spectroscopy of electrically triggered exploding nanoparticle analytes on graphene/SiO2/Si substrate.” Journal of Vacuum Science & Technology B, Nanotechnology and Microelectronics: Materials, Processing, Measurement, and Phenomena, vol. 34, no. 6, 2016, doi:10.1116/1.4964819.
- Sattler, Klaus D. Carbon nanomaterials sourcebook. Vol. 1, CRC Press, 2016.
- Politou, Maria, et al. “Evaluation of multilayer graphene for advanced interconnects.” Microelectronic Engineering, vol. 167, 5 Jan. 2017, pp. 1–5., doi:10.1016/j.mee.2016.09.011.
- Hwang, Michael T., et al. “Highly specific SNP detection using 2D graphene electronics and DNA strand displacement.” Proceedings of the National Academy of Sciences, vol. 113, no. 26, 2016, pp. 7088–7093., doi:10.1073/pnas.1603753113.
- Nieto, Andy. “Graphene reinforced metal and ceramic matrix composites: a review.” International Materials Reviews, vol. 62, no. 5, 27 Oct. 2017, pp. 241–302., doi:10.1080/09506608.2016.1219481.
- Khorasaninejad, M., et al. “Highly Enhanced Raman Scattering of Graphene using Plasmonic Nano-Structure.” Scientific Reports, vol. 3, no. 1, 2013, doi:10.1038/srep02936.
- Kunz, Daniel A., et al. “Space-Resolved In-Plane Moduli of Graphene Oxide and Chemically Derived Graphene Applying a Simple Wrinkling Procedure (Adv. Mater. 9/2013).” Advanced Materials, vol. 25, no. 9, Apr. 2013, pp. 1336–1336., doi:10.1002/adma.201370060.
- Peng, Shuhua, et al. “Microwetting of Supported Graphene on Hydrophobic Surfaces Revealed by Polymerized Interfacial Femtodroplets.” Langmuir, vol. 30, no. 33, 2014, pp. 10043–10049., doi:10.1021/la5022774.
- Luo, Wen, et al. “Single-Layer Graphene as an Effective Mediator of the Metal–Support Interaction.” The Journal of Physical Chemistry Letters, vol. 5, no. 11, 2014, pp. 1837–1844., doi:10.1021/jz500425j.
- Suemoto, Tohru, et al. “Thickness dependent hot-Phonon effects observed by femtosecond mid-Infrared luminescence in graphene.” 19th International Conference on Ultrafast Phenomena, 2014, doi:10.1364/up.2014.07.mon.p1.32.
- Zhou, Haosen, et al. “Influence of CO2 On the Stability of Discharge Performance for Li-Air Battery with Hybrid Electrolyte Based On the Graphene Sheet.” Conference: 224th ECS Meeting. Oct. 2013, doi:10.1039/c3ra47258b.
- Thareja, Vrinda. “Electrically Tunable Optical Absorption in a Graphene-Based Salisbury Screen.” CORNELL UNIVERSITY LIBRARY, 7 Sept. 2014.
- Horvath, Cameron S. “Light Propagation and Photothermal Nonlinearity in Graphene-Si Waveguides .” University of Alberta Libraries , Nov. 2013, doi:10.7939/R3ST1N.
- Li, Cheng, et al. “Interference characteristics in a Fabry–Perot cavity with graphene membrane for optical fiber pressure sensors.” Microsystem Technologies, vol. 21, no. 11, 2014, pp. 2297–2306., doi:10.1007/s00542-014-2333-2.
- Kou, Jun-Long, et al. “Platform for enhanced light–graphene interaction length and miniaturizing fiber stereo devices.” Optica, vol. 1, no. 5, 2014, p. 307., doi:10.1364/optica.1.000307.
- Karnik, Rohit. “Ionic and Molecular Transport Through Graphene Membranes.” Transport and Reactivity of Solutions in Confined Hydrosystems NATO Science for Peace and Security Series C: Environmental Security, Dec. 2013, pp. 95–102., doi:10.1007/978-94-007-7534-3_8.
- Warren, A. J., et al. “In vacuo X-Ray data collection from graphene-Wrapped protein crystals.” Acta Crystallographica Section D STRUCTURAL BIOLOGY, vol. D71, 2015, pp. 2079–2088., doi:10.1107/S1399004715014194.
- Wen, Chenyu, et al. “Assessing kinetics of surface adsorption–desorption of gas molecules via electrical measurements.” Sensors and Actuators B: Chemical, vol. 223, 2016, pp. 791–798., doi:10.1016/j.snb.2015.10.019.
- Li, Cheng, et al. “Analyzing the applicability of miniature ultra-High sensitivity Fabry–Perot acoustic sensor using a nanothick graphene diaphragm.” Measurement Science and Technology, vol. 26, no. 8, 10 July 2015.
- Li, Cheng, et al. “Analyzing the temperature sensitivity of Fabry-Perot sensor using multilayer graphene diaphragm.” OSA Publishing, vol. 23, no. 21, 2015, pp. 27494–27502., doi:10.1364/OE.23.027494.
- Lau, K. Y., et al. “High Signal-To-Noise Ratio Q-Switching Erbium Doped Fiber Laser Pulse Emission Utilizing Single Layer Trivial Transfer Graphene Film Saturable Absorber.” Jurnal Teknologi, vol. 78, no. 3, 2016, doi:10.11113/jt.v78.7478.
- Zheng, Shijun, et al. “Acoustic charge transport induced by the surface acoustic wave in chemical doped graphene.” Applied Physics Letters, vol. 109, no. 18, 2016, p. 183110., doi:10.1063/1.4967192.
- Deng, Xiangquan, et al. “Terahertz-Induced photothermoelectric response in graphene-Metal contact structures.” Journal of Physics D: Applied Physics, vol. 49, no. 42, 2016, p. 425101., doi:10.1088/0022-3727/49/42/425101.
- Luo, Wen, and Spyridon Zafeiratos. “Inside Back Cover: Graphene-Coated ZnO and SiO2 as Supports for CoO Nanoparticles with Enhanced Reducibility.” ChemPhysChem, vol. 17, no. 19, Apr. 2016, pp. 3146–3146., doi:10.1002/cphc.201601023.
- Lau, K.Y., et al. “Passively mode-Locked soliton femtosecond pulses employing graphene saturable absorber.” Optics & Laser Technology, vol. 94, 2017, pp. 221–227., doi:10.1016/j.optlastec.2017.03.035.
- Fitri, Meika Aidil, et al. “Fabrication of TiO 2 -Graphene photocatalyst by direct chemical vapor deposition and its anti-Fouling property.” Materials Chemistry and Physics, vol. 198, 2017, pp. 42–48., doi:10.1016/j.matchemphys.2017.05.053.
- Li, Cheng, et al. “Nondestructive andin situdetermination of graphene layers using optical fiber Fabry–Perot interference.” Measurement Science and Technology, vol. 28, no. 2, Dec. 2017, p. 025206., doi:10.1088/1361-6501/aa54f8.
- Gao, Xiangyang, et al. “Measuring Graphene Adhesion on Silicon Substrate by Single and Dual Nanoparticle-Loaded Blister.” Advanced Materials Interfaces, vol. 4, no. 9, 2017, p. 1601023., doi:10.1002/admi.201601023.
- Tang, Xiaoduan, et al. “Five Orders of Magnitude Reduction in Energy Coupling across Corrugated Graphene/Substrate Interfaces.” ACS Applied Materials & Interfaces, vol. 6, no. 4, July 2014, pp. 2809–2818., doi:10.1021/am405388a.
- Morrow, W. K., et al. “(Invited) The Use of Graphene as a Solid State Diffusion Barrier.” ECS Transactions, vol. 61, no. 4, 2014, pp. 371–379., doi:10.1149/06104.0371ecst.
- Zheng, Haisheng, et al. “Ultrafine Pt nanoparticle induced doping/Strain of single layer graphene: experimental corroboration between conduction and Raman characteristics.” Journal of Materials Science: Materials in Electronics, vol. 26, no. 7, 2015, pp. 4746–4753., doi:10.1007/s10854-015-3043-y.
- Blinco , James P, et al. “Spin-Coated Carbon." Chemical Science, no. 9, 2013, pp. 3411–3415., doi:10.1039/C3SC51396C.
- Zheng, H., et al. “Effect of Sub 1-Nm Pt Nanoparticle on the Conduction Properties of Graphene Based Field Effect Transistor.” ECS Transactions, vol. 61, no. 39, Jan. 2014, pp. 1–11., doi:10.1149/06139.0001ecst.
- Mckitterick, Christopher B., et al. “Electron-Phonon cooling in large monolayer graphene devices.” Physical Review B, vol. 93, no. 7, Apr. 2016, doi:10.1103/physrevb.93.075410.
- Chan, Chun Yu. “Graphene based electrical biosensors for the detection of biomolecules.” The Hong Kong Polytechnic University, 2016.
- Pápa, Z., et al. “Spectroscopic ellipsometric investigation of graphene and thin carbon films from the point of view of depolarization effects.” Applied Surface Science, vol. 421, 2017, pp. 714–721., doi:10.1016/j.apsusc.2016.11.231.
- Hussain, Mushtaque, et al. “The improved piezoelectric properties of ZnO nanorods with oxygen plasma treatment on the single layer graphene coated polymer substrate.” Physica status solidi (a), vol. 211, no. 2, Oct. 2014, pp. 455–459., doi:10.1002/pssa.201300330.
- Kafiah, Feras, et al. “Synthesis of Graphene Based Membranes: Effect of Substrate Surface Properties on Monolayer Graphene Transfer.” Materials, vol. 10, no. 1, 2017, p. 86., doi:10.3390/ma10010086.
- Liang, Ji, et al. “Modulation of acousto-Electric current using a hybrid on-Chip AlN SAW/GFET device.” Applied Physics Letters, vol. 110, no. 24, Dec. 2017, p. 243504., doi:10.1063/1.4986481.
- Zhang, Yingjie & Kim, Youngseok & J. Gilbert, Matthew & Mason, Nadya. (2017). Electron transport in strain superlattices of graphene.
- Yan, Xiao-Qing, et al. “Polarization dependence of graphene transient optical response: interplay between incident direction and anisotropic distribution of nonequilibrium carriers.” Journal of the Optical Society of America B, vol. 34, no. 1, 2016, p. 218., doi:10.1364/josab.34.000218.
- Huang, Kun, et al. “Graphene coupled with Pt cubic nanoparticles for high performance, air-Stable graphene-Silicon solar cells.” Nano Energy, vol. 32, 2017, pp. 225–231., doi:10.1016/j.nanoen.2016.12.042.
- Lu, Bingyu, et al. “Roughened cylindrical gold layer with curve graphene coating for enhanced sensitivity of fiber SPR sensor.” 2017 19th International Conference on Solid-State Sensors, Actuators and Microsystems (TRANSDUCERS), 2017, doi:10.1109/transducers.2017.7994461.
- Kafiah, Feras, et al. “Synthesis of Graphene Based Membranes: Effect of Substrate Surface Properties on Monolayer Graphene Transfer.” Materials, vol. 10, no. 1, 2017, p. 86., doi:10.3390/ma10010086.
- Hsu, Wei-Hao, et al. “Low-Energy electron point projection microscopy/Diffraction study of suspended graphene.” Applied Surface Science, vol. 423, 30 Nov. 2017, pp. 266–274., doi:10.1016/j.apsusc.2017.06.148.
- Peng, Shuhua, et al. “Microwetting of Supported Graphene on Hydrophobic Surfaces Revealed by Polymerized Interfacial Femtodroplets.” Langmuir, vol. 30, no. 33, 2014, pp. 10043–10049., doi:10.1021/la5022774.
- Horvath, Cameron, et al. “Edge-Conformed silicon-Graphene waveguides: Fabrication and measurements.” 11th International Conference on Group IV Photonics (GFP), 2014, doi:10.1109/group4.2014.6961953.
- Watanabe, Hiroshi, et al. “Femtosecond midi-Infrared luminescence with hot-Phonon effects in graphenes and graphite.”
- Hassan, Saad. Transparent conductive article.
- Liang, Ji, et al. “Manipulation of carriers in graphene using an on-Chip acoustic wave device.” 2017 IEEE International Ultrasonics Symposium (IUS), 2017, doi:10.1109/ultsym.2017.8092898.
- Luo, Wen, et al. “Interaction of bimetallic PtCo layers with bare and graphene-Covered ZnO(0001) supports.” Surface Science, vol. 669, 2018, pp. 64–70., doi:10.1016/j.susc.2017.11.001.
- Jiang, Wen-Shuai, et al. “Preparation of high-Quality graphene using triggered microwave reduction under an air atmosphere.” Journal of Materials Chemistry C, 2018, doi:10.1039/c7tc03957c.
- Dong, Nannan, et al. “Pressure and Temperature Sensor Based on Graphene Diaphragm and Fiber Bragg Gratings.” IEEE Photonics Technology Letters, 2017, pp. 1–1., doi:10.1109/lpt.2017.2786292.
- Huczko, Andrzej, et al. “Efficient one-Pot combustion synthesis of few-Layered graphene.” Physica status solidi (b), vol. 252, no. 11, 2015, pp. 2412–2417., doi:10.1002/pssb.201552233.
- Lu, Bingyu, et al. “Roughened cylindrical gold layer with curve graphene coating for enhanced sensitivity of fiber SPR sensor.” 2017 19th International Conference on Solid-State Sensors, Actuators and Microsystems (TRANSDUCERS), 2017, doi:10.1109/transducers.2017.7994461.
- Fitri, Meika Aidil, et al. “Fabrication of TiO 2 -Graphene photocatalyst by direct chemical vapor deposition and its anti-Fouling property.” Materials Chemistry and Physics, vol. 198, 2017, pp. 42–48., doi:10.1016/j.matchemphys.2017.05.053.
- Bezryadin, A, et al. “Large energy storage efficiency of the dielectric layer of graphene nanocapacitors.” Nanotechnology, vol. 28, no. 49, 2017, p. 495401., doi:10.1088/1361-6528/aa935c.
- Shi, Cheng, et al. “Metamaterial-Based graphene thermal emitter.” Nano Research, June 2017, doi:10.1007/s12274-017-1922-7.
- McCaffrey, Debra L., et al. “Mechanism of ion adsorption to aqueous interfaces: Graphene/Water vs. air/Water.” Proceedings of the National Academy of Sciences, vol. 114, no. 51, 2017, pp. 13369–13373., doi:10.1073/pnas.1702760114.
- Zhang, Qin, et al. “Negative differential resistance and hysteresis in graphene-Based organic light-Emitting devices.” Journal of Materials Chemistry C, The Royal Society of Chemistry, 1 Jan. 2018, pubs.rsc.org/en/Content/ArticleLanding/2017/TC/C7TC05148D#!
- Miskin, Marc Z., et al. “Graphene-Based bimorphs for micron-Sized, autonomous origami machines.” Proceedings of the National Academy of Sciences, vol. 115, no. 3, Feb. 2018, pp. 466–470., doi:10.1073/pnas.1712889115.
- Bukola, Saheed, et al. “Selective Proton/Deuteron Transport through Nafion|Graphene|Nafion Sandwich Structures at High Current Density.” Journal of the American Chemical Society, vol. 140, no. 5, 2018, pp. 1743–1752., doi:10.1021/jacs.7b10853.
- Brown, Morgan A., et al. “Graphene Biotransistor Interfaced with a Nitrifying Biofilm.” Environmental Science & Technology Letters, vol. 2, no. 4, Mar. 2015, pp. 118–122., doi:10.1021/acs.estlett.5b00025.
- Woo, Sung Oh, and Winfried Teizer. “The effect of electron induced hydrogenation of graphene on its electrical transport properties.” Applied Physics Letters, vol. 103, no. 4, 2013, p. 041603., doi:10.1063/1.4816475.
- Li, Cheng, et al. “Manipulation of Nonlinear Optical Properties of Graphene Bonded Fiber Devices by Thermally Engineering Fermi-Dirac Distribution.” Advanced Optical Materials, vol. 5, no. 21, 2017, doi:10.1002/adom.201770103.
- Chirayath, V. A, et al. “Positron induced electron emission from graphene.” IOP Science, Journal of Physics: Conference Series.
- Lock, Evgeniya H., et al. Stable IR Transparent Conductive Graphene Hybrid Materials and Methods of Making.
- Beechem, Thomas E., et al. “Self-Heating and Failure in Scalable Graphene Devices.” Scientific Reports, vol. 6, no. 1, Sept. 2016, doi:10.1038/srep26457.
- Ma, Yufeng, and Anni Siitonen. Chemical sensor using molecularly-Imprinted single layer graphene.
- Morrow, Wayne K., et al. “Role of graphene interlayers in mitigating degradation of Ni/Au ohmic contact morphology on p-Type GaN.” Vacuum, vol. 128, 2016, pp. 34–38., doi:10.1016/j.vacuum.2016.03.004.
- Nagamanasa, Kandula Hima, et al. “Liquid-Cell Electron Microscopy of Adsorbed Polymers.” Advanced Materials, vol. 29, no. 41, 2017, p. 1703555., doi:10.1002/adma.201703555.
- Hui, Fei. “Variability of graphene devices fabricated using graphene inks: Atomic force microscope tips.” Surface and Coatings Technology, vol. 320, 25 June 2017, doi:10.1016/j.surfcoat.2016.12.020.
- Chirayath, V A, et al. “Investigation of graphene using low energy positron annihilation induced Doppler broadening spectroscopy.” Journal of Physics: Conference Series, vol. 791, 2017, p. 012032., doi:10.1088/1742-6596/791/1/012032.
- Niu, Tianxiao, et al. “Indentation behavior of the stiffest membrane mounted on a very compliant substrate: Graphene on PDMS.” International Journal of Solids and Structures, vol. 132-133, 2018, pp. 1–8., doi:10.1016/j.ijsolstr.2017.05.038.
- Nashed, Ramy, et al. “Ultra-High Mobility in Dielectrically Pinned CVD Graphene.” IEEE Journal of the Electron Devices Society, vol. 4, no. 6, 2016, pp. 466–472., doi:10.1109/jeds.2016.2595498.
- Niu, Tianxiao, et al. “Fracture behavior of graphene mounted on stretchable substrate.” Carbon, vol. 109, 2016, pp. 852–859., doi:10.1016/j.carbon.2016.08.087.
- Boutilier, Michael S H, et al. “Knudsen effusion through polymer-Coated three-Layer porous graphene membranes.” Nanotechnology, vol. 28, no. 18, Oct. 2017, p. 184003., doi:10.1088/1361-6528/aa680f.
- Du, Feng, et al. “Surface stress of graphene layers supported on soft substrate.” Scientific Reports, vol. 6, no. 1, Nov. 2016, doi:10.1038/srep25653.
- Yokaribas, Volkan, et al. “Strain Gauges Based on CVD Graphene Layers and Exfoliated Graphene Nanoplatelets with Enhanced Reproducibility and Scalability for Large Quantities.” Sensors, vol. 17, no. 12, 2017, p. 2937., doi:10.3390/s17122937.
- Kuru, Cihan, et al. “Enhanced Power Conversion Efficiency of Graphene/Silicon Heterojunction Solar Cells Through NiO Induced Doping.” Journal of Nanoscience and Nanotechnology, vol. 16, no. 1, Jan. 2016, pp. 1190–1193., doi:10.1166/jnn.2016.12079.
|
-
Research Citations of ACS Material Products
- Chen, Ruiyi, et al. “Co-Percolating Graphene-Wrapped Silver Nanowire Network for High Performance, Highly Stable, Transparent Conducting Electrodes.” Advanced Functional Materials, vol. 23, no. 41, 2013, pp. 5150–5158., doi:10.1002/adfm.201300124.
- O’Hern, Sean C., et al. “Selective Molecular Transport through Intrinsic Defects in a Single Layer of CVD Graphene.” ACS Nano, vol. 6, no. 11, Sept. 2012, pp. 10130–10138., doi:10.1021/nn303869m.
- Yoo, Jae-Hyuck, et al. “Graphene folds by femtosecond laser ablation.” Applied Physics Letters, vol. 100, no. 23, Apr. 2012, p. 233124., doi:10.1063/1.4724213.
- Longchamp, Jean-Nicolas, et al. “Low-Energy electron transmission imaging of clusters on free-Standing graphene.” Applied Physics Letters, vol. 101, no. 11, Oct. 2012, p. 113117., doi:10.1063/1.4752717.
- Chen, Xu-Dong, et al. “High-Quality and efficient transfer of large-Area graphene films onto different substrates.” Carbon, vol. 56, May 2013, pp. 271–278., doi:10.1016/j.carbon.2013.01.011.
- Ye, Qing., et al. “Polarization-Dependent optical absorption of graphene under total internal reflection.” Applied Physics Letters, vol. 102, no. 2, doi:10.1063/1.4776694.
- Longchamp, Jean-Nicolas, et al. “Ultraclean freestanding graphene by platinum-Metal catalysis.” Journal of Vacuum Science & Technology B, Nanotechnology and Microelectronics: Materials, Processing, Measurement, and Phenomena, vol. 31, no. 2, 2013, p. 020605., doi:10.1116/1.4793746.
- Wang, Yung Yu, and Peter J. Burke. “A large-Area and contamination-Free graphene transistor for liquid-Gated sensing applications.” Applied Physics Letters, vol. 103, no. 5, 2013, p. 052103., doi:10.1063/1.4816764.
- Wang, Peng, et al. “Accurate layers determination of graphene on transparent substrate based on polarization-Sensitive absorption effect.” Applied Physics Letters, vol. 103, no. 18, 2013, p. 181902., doi:10.1063/1.4827812.
- Zhou, Rong, et al. “Large-Energy, narrow-Bandwidth laser pulse at 1645 nm in a diode-Pumped Er:YAG solid-State laser passively Q-Switched by a monolayer graphene saturable absorber.” Applied Optics, vol. 53, no. 2, Sept. 2014, p. 254., doi:10.1364/ao.53.000254.
- Choi, Duyoung, et al. “Nanopatterned Graphene Field Effect Transistor Fabricated Using Block Co-Polymer Lithography.” Materials Research Letters, vol. 2, no. 3, Sept. 2014, pp. 131–139., doi:10.1080/21663831.2013.876676.
- Srisonphan, Siwapon, et al. “Space charge neutralization by electron-Transparent suspended graphene.” Scientific Reports, vol. 4, no. 1, 2014, doi:10.1038/srep03764.
- Joiner, C. A., et al. “Cleaning graphene with a titanium sacrificial layer.” Applied Physics Letters, vol. 104, no. 22, Feb. 2014, p. 223109., doi:10.1063/1.4881886.
- Roy, T., et al. “Tunneling characteristics in chemical vapor deposited graphene–hexagonal boron nitride–graphene junctions.” Applied Physics Letters, vol. 104, no. 12, 2014, p. 123506., doi:10.1063/1.4870073.
- Burke, Peter J. “Charging the Quantum Capacitance of Graphene with a Single Biological Ion Channel.” Biophysical Journal, vol. 106, no. 2, 2014, doi:10.1016/j.bpj.2013.11.2796.
- Li, Peining, et al. “Graphene-Enhanced Infrared Near-Field Microscopy.” Nano Letters, vol. 14, no. 8, 2014, pp. 4400–4405., doi:10.1021/nl501376a.
- Boutilier, Michael S. H., et al. “Implications of Permeation through Intrinsic Defects in Graphene on the Design of Defect-Tolerant Membranes for Gas Separation.” ACS Nano, vol. 8, no. 1, Mar. 2014, pp. 841–849., doi:10.1021/nn405537u.
- Lee, Jiye, et al. “Switching Individual Quantum Dot Emission through Electrically Controlling Resonant Energy Transfer to Graphene.” Nano Letters, vol. 14, no. 12, 2014, pp. 7115–7119., doi:10.1021/nl503587z.
- Srisonphan, Siwapon, and Komsan Hongesombut. “Tuning the ballistic electron transport of spatial graphene–metal sandwich electrode on a vacuum-Silicon-Based device.” RSC Advances, vol. 5, no. 3, 2015, pp. 2032–2037., doi:10.1039/c4ra09503k.
- Hui, Fei, et al. “Mechanical properties of locally oxidized graphene electrodes.” Archive of Applied Mechanics, vol. 85, no. 3, 2014, pp. 339–345., doi:10.1007/s00419-014-0957-4.
- Fujimoto, A, et al. “Negative magnetoresistance in Ti-Cleaned single-Layer graphene.” Journal of Physics: Conference Series, vol. 603, 2015, p. 012021., doi:10.1088/1742-6596/603/1/012021.
- Thareja, Vrinda, et al. “Electrically Tunable Coherent Optical Absorption in Graphene with Ion Gel.” Nano Letters, vol. 15, no. 3, 2015, pp. 1570–1576., doi:10.1021/nl503431d.
- Ye, X. H., et al. “Corrosion resistance of graphene directly and locally grown on bulk nickel substrate by laser irradiation.” RSC Advances, vol. 5, no. 45, 2015, pp. 35384–35390., doi:10.1039/c5ra01267h.
- Horvath, Cameron . “Fabrication and Characterization of Edge-Conformed Graphene-Silicon Waveguides.” IEEE Photonics Technology Letters, vol. 27, no. 6, pp. 585–587., doi:10.1109/LPT.2014.2385757.
- Marta, Bogdan, et al. “Efficient etching-Free transfer of high quality, large-Area CVD grown graphene onto polyvinyl alcohol films.” Applied Surface Science, vol. 363, 2016, pp. 613–618., doi:10.1016/j.apsusc.2015.11.265.
- Zheng, Guanpeng, et al. “Improved Transfer Quality of CVD-Grown Graphene by Ultrasonic Processing of Target Substrates: Applications for Ultra-Fast Laser Photonics.” ACS Applied Materials & Interfaces, vol. 5, no. 20, Sept. 2013, pp. 10288–10293., doi:10.1021/am403205v.
- Zeng, Yong, et al. “Investigate the interface structure and growth mechanism of high quality ZnO films grown on multilayer graphene layers.” Applied Surface Science, vol. 301, 2014, pp. 391–395., doi:10.1016/j.apsusc.2014.02.088.
- Ye, Qing, et al. “Polarization-Dependent optical absorption of graphene under total internal reflection.” Applied Physics Letters, vol. 102, no. 2, Jan. 2013, doi:10.1063/1.4776694.
- Watanabe, Hiroshi, et al. “Layer number dependence of carrier lifetime in graphenes observed using time-Resolved mid-Infrared luminescence.” Chemical Physics Letters, vol. 637, 2015, pp. 58–62., doi:10.1016/j.cplett.2015.07.046.
- He, Yingbo, et al. “Strongly enhanced Raman scattering of graphene by a single gold nanorod.” Applied Physics Letters, vol. 107, no. 5, Mar. 2015, p. 053104., doi:10.1063/1.4927759.
- Choi, Duyoung, et al. “Uniformly Nanopatterned Graphene Field-Effect Transistors with Enhanced Properties.” Nanoscale Research Letters, vol. 10, no. 1, Nov. 2015, doi:10.1186/s11671-015-0976-2.
- Miao, Lili, et al. “Broadband ultrafast nonlinear optical response of few-Layers graphene: toward the mid-Infrared regime.” Photonics Research, vol. 3, no. 5, 2015, p. 214., doi:10.1364/prj.3.000214.
- Hu, Jianchen, et al. “Improvement of the electrical contact resistance at rough interfaces using two dimensional materials.” Journal of Applied Physics, vol. 118, no. 21, July 2015, p. 215301., doi:10.1063/1.4936366.
- Jain, Tarun, et al. “Heterogeneous sub-Continuum ionic transport in statistically isolated graphene nanopores.” Nature Nanotechnology, vol. 10, no. 12, May 2015, pp. 1053–1057., doi:10.1038/nnano.2015.222.
- Srisonphan, Siwapon, and Komsan Hongesombut. “Tuning the ballistic electron transport of spatial graphene–metal sandwich electrode on a vacuum-Silicon-Based device.” RSC Advances, vol. 5, no. 3, 2015, pp. 2032–2037., doi:10.1039/c4ra09503k.
- Liu, Xiangjiang, et al. “Compact Shielding of Graphene Monolayer Leads to Extraordinary SERS-Active Substrate with Large-Area Uniformity and Long-Term Stability.” Scientific Reports, vol. 5, no. 1, 2015, doi:10.1038/srep17167.
- Hui, Fei, et al. “Moving graphene devices from lab to market: advanced graphene-Coated nanoprobes.” Nanoscale, vol. 8, no. 16, 2016, pp. 8466–8473., doi:10.1039/c5nr06235g.
- Ye, Xiaohui, et al. “Protecting carbon steel from corrosion by laser in situ grown graphene films.” Carbon, vol. 94, 2015, pp. 326–334., doi:10.1016/j.carbon.2015.06.080.
- Wu, Man, et al. “Wavelength switchable graphene Q-Switched fiber laser with cascaded fiber Bragg gratings.” Optics Communications, vol. 368, 2016, pp. 81–85., doi:10.1016/j.optcom.2016.01.069.
- Mackin, Charles, and Tomás Palacios. “Large-Scale sensor systems based on graphene electrolyte-Gated field-Effect transistors.” The Analyst, vol. 141, no. 9, 2016, pp. 2704–2711., doi:10.1039/c5an02328a.
- Politou, Maria, et al. “Multi-Layer graphene interconnect.” 2016 IEEE International Interconnect Technology Conference / Advanced Metallization Conference (IITC/AMC), July 2016, doi:10.1109/IITC-AMC.2016.7507731.
- Cao, Zhengmin, et al. “Nano-Gap between a gold tip and nanorod for polarization dependent surface enhanced Raman scattering.” Applied Physics Letters, vol. 109, no. 23, May 2016, p. 233103., doi:10.1063/1.4971832.
- Deng, Xiangquan, et al. “Terahertz-Induced photothermoelectric response in graphene-Metal contact structures.” Journal of Physics D: Applied Physics, vol. 49, no. 42, 2016, p. 425101., doi:10.1088/0022-3727/49/42/425101.
- Guo, Chu-Cai, et al. “Experimental Demonstration of Total Absorption over 99% in the Near Infrared for Monolayer-Graphene-Based Subwavelength Structures.” Advanced Optical Materials, vol. 4, no. 12, Jan. 2016, pp. 1955–1960., doi:10.1002/adom.201600481.
- Hu, Jianbo, et al. “Rippling ultrafast dynamics of suspended 2D monolayers, graphene.” Proceedings of the National Academy of Sciences, vol. 113, no. 43, Oct. 2016, doi:10.1073/pnas.1613818113.
- Li, Cheng, et al. “Measurement of the Adhesion Energy of Pressurized Graphene Diaphragm Using Optical Fiber Fabry–Perot Interference.” IEEE Sensors Journal, vol. 16, no. 10, 2016, pp. 3664–3669., doi:10.1109/jsen.2016.2536783.
- Yuan, Jun, et al. “Modulation of far-Infrared light transmission by graphene-Silicon Schottky junction.” Optical Materials Express, vol. 6, no. 12, 2016, p. 3908., doi:10.1364/ome.6.003908.
- Li, Cheng, et al. “Measurement of thermal expansion coefficient of graphene diaphragm using optical fiber Fabry–Perot interference.” Measurement Science and Technology, vol. 27, no. 7, 2016, p. 075102., doi:10.1088/0957-0233/27/7/075102.
- Kafiah, Feras M., et al. “Monolayer graphene transfer onto polypropylene and polyvinylidenedifluoride microfiltration membranes for water desalination.” Desalination, vol. 388, 2016, pp. 29–37., doi:10.1016/j.desal.2016.02.027.
- Wu, Xiangyu, et al. “Doping of graphene for the application in nano-Interconnect.” Microelectronic Engineering, vol. 167, 5 Jan. 2017, pp. 42–46., doi:10.1016/j.mee.2016.10.013.
- Liu, Siyang, et al. “Atomic emission spectroscopy of electrically triggered exploding nanoparticle analytes on graphene/SiO2/Si substrate.” Journal of Vacuum Science & Technology B, Nanotechnology and Microelectronics: Materials, Processing, Measurement, and Phenomena, vol. 34, no. 6, 2016, doi:10.1116/1.4964819.
- Sattler, Klaus D. Carbon nanomaterials sourcebook. Vol. 1, CRC Press, 2016.
- Politou, Maria, et al. “Evaluation of multilayer graphene for advanced interconnects.” Microelectronic Engineering, vol. 167, 5 Jan. 2017, pp. 1–5., doi:10.1016/j.mee.2016.09.011.
- Hwang, Michael T., et al. “Highly specific SNP detection using 2D graphene electronics and DNA strand displacement.” Proceedings of the National Academy of Sciences, vol. 113, no. 26, 2016, pp. 7088–7093., doi:10.1073/pnas.1603753113.
- Nieto, Andy. “Graphene reinforced metal and ceramic matrix composites: a review.” International Materials Reviews, vol. 62, no. 5, 27 Oct. 2017, pp. 241–302., doi:10.1080/09506608.2016.1219481.
- Khorasaninejad, M., et al. “Highly Enhanced Raman Scattering of Graphene using Plasmonic Nano-Structure.” Scientific Reports, vol. 3, no. 1, 2013, doi:10.1038/srep02936.
- Kunz, Daniel A., et al. “Space-Resolved In-Plane Moduli of Graphene Oxide and Chemically Derived Graphene Applying a Simple Wrinkling Procedure (Adv. Mater. 9/2013).” Advanced Materials, vol. 25, no. 9, Apr. 2013, pp. 1336–1336., doi:10.1002/adma.201370060.
- Peng, Shuhua, et al. “Microwetting of Supported Graphene on Hydrophobic Surfaces Revealed by Polymerized Interfacial Femtodroplets.” Langmuir, vol. 30, no. 33, 2014, pp. 10043–10049., doi:10.1021/la5022774.
- Luo, Wen, et al. “Single-Layer Graphene as an Effective Mediator of the Metal–Support Interaction.” The Journal of Physical Chemistry Letters, vol. 5, no. 11, 2014, pp. 1837–1844., doi:10.1021/jz500425j.
- Suemoto, Tohru, et al. “Thickness dependent hot-Phonon effects observed by femtosecond mid-Infrared luminescence in graphene.” 19th International Conference on Ultrafast Phenomena, 2014, doi:10.1364/up.2014.07.mon.p1.32.
- Zhou, Haosen, et al. “Influence of CO2 On the Stability of Discharge Performance for Li-Air Battery with Hybrid Electrolyte Based On the Graphene Sheet.” Conference: 224th ECS Meeting. Oct. 2013, doi:10.1039/c3ra47258b.
- Thareja, Vrinda. “Electrically Tunable Optical Absorption in a Graphene-Based Salisbury Screen.” CORNELL UNIVERSITY LIBRARY, 7 Sept. 2014.
- Horvath, Cameron S. “Light Propagation and Photothermal Nonlinearity in Graphene-Si Waveguides .” University of Alberta Libraries , Nov. 2013, doi:10.7939/R3ST1N.
- Li, Cheng, et al. “Interference characteristics in a Fabry–Perot cavity with graphene membrane for optical fiber pressure sensors.” Microsystem Technologies, vol. 21, no. 11, 2014, pp. 2297–2306., doi:10.1007/s00542-014-2333-2.
- Kou, Jun-Long, et al. “Platform for enhanced light–graphene interaction length and miniaturizing fiber stereo devices.” Optica, vol. 1, no. 5, 2014, p. 307., doi:10.1364/optica.1.000307.
- Karnik, Rohit. “Ionic and Molecular Transport Through Graphene Membranes.” Transport and Reactivity of Solutions in Confined Hydrosystems NATO Science for Peace and Security Series C: Environmental Security, Dec. 2013, pp. 95–102., doi:10.1007/978-94-007-7534-3_8.
- Warren, A. J., et al. “In vacuo X-Ray data collection from graphene-Wrapped protein crystals.” Acta Crystallographica Section D STRUCTURAL BIOLOGY, vol. D71, 2015, pp. 2079–2088., doi:10.1107/S1399004715014194.
- Wen, Chenyu, et al. “Assessing kinetics of surface adsorption–desorption of gas molecules via electrical measurements.” Sensors and Actuators B: Chemical, vol. 223, 2016, pp. 791–798., doi:10.1016/j.snb.2015.10.019.
- Li, Cheng, et al. “Analyzing the applicability of miniature ultra-High sensitivity Fabry–Perot acoustic sensor using a nanothick graphene diaphragm.” Measurement Science and Technology, vol. 26, no. 8, 10 July 2015.
- Li, Cheng, et al. “Analyzing the temperature sensitivity of Fabry-Perot sensor using multilayer graphene diaphragm.” OSA Publishing, vol. 23, no. 21, 2015, pp. 27494–27502., doi:10.1364/OE.23.027494.
- Lau, K. Y., et al. “High Signal-To-Noise Ratio Q-Switching Erbium Doped Fiber Laser Pulse Emission Utilizing Single Layer Trivial Transfer Graphene Film Saturable Absorber.” Jurnal Teknologi, vol. 78, no. 3, 2016, doi:10.11113/jt.v78.7478.
- Zheng, Shijun, et al. “Acoustic charge transport induced by the surface acoustic wave in chemical doped graphene.” Applied Physics Letters, vol. 109, no. 18, 2016, p. 183110., doi:10.1063/1.4967192.
- Deng, Xiangquan, et al. “Terahertz-Induced photothermoelectric response in graphene-Metal contact structures.” Journal of Physics D: Applied Physics, vol. 49, no. 42, 2016, p. 425101., doi:10.1088/0022-3727/49/42/425101.
- Luo, Wen, and Spyridon Zafeiratos. “Inside Back Cover: Graphene-Coated ZnO and SiO2 as Supports for CoO Nanoparticles with Enhanced Reducibility.” ChemPhysChem, vol. 17, no. 19, Apr. 2016, pp. 3146–3146., doi:10.1002/cphc.201601023.
- Lau, K.Y., et al. “Passively mode-Locked soliton femtosecond pulses employing graphene saturable absorber.” Optics & Laser Technology, vol. 94, 2017, pp. 221–227., doi:10.1016/j.optlastec.2017.03.035.
- Fitri, Meika Aidil, et al. “Fabrication of TiO 2 -Graphene photocatalyst by direct chemical vapor deposition and its anti-Fouling property.” Materials Chemistry and Physics, vol. 198, 2017, pp. 42–48., doi:10.1016/j.matchemphys.2017.05.053.
- Li, Cheng, et al. “Nondestructive andin situdetermination of graphene layers using optical fiber Fabry–Perot interference.” Measurement Science and Technology, vol. 28, no. 2, Dec. 2017, p. 025206., doi:10.1088/1361-6501/aa54f8.
- Gao, Xiangyang, et al. “Measuring Graphene Adhesion on Silicon Substrate by Single and Dual Nanoparticle-Loaded Blister.” Advanced Materials Interfaces, vol. 4, no. 9, 2017, p. 1601023., doi:10.1002/admi.201601023.
- Tang, Xiaoduan, et al. “Five Orders of Magnitude Reduction in Energy Coupling across Corrugated Graphene/Substrate Interfaces.” ACS Applied Materials & Interfaces, vol. 6, no. 4, July 2014, pp. 2809–2818., doi:10.1021/am405388a.
- Morrow, W. K., et al. “(Invited) The Use of Graphene as a Solid State Diffusion Barrier.” ECS Transactions, vol. 61, no. 4, 2014, pp. 371–379., doi:10.1149/06104.0371ecst.
- Zheng, Haisheng, et al. “Ultrafine Pt nanoparticle induced doping/Strain of single layer graphene: experimental corroboration between conduction and Raman characteristics.” Journal of Materials Science: Materials in Electronics, vol. 26, no. 7, 2015, pp. 4746–4753., doi:10.1007/s10854-015-3043-y.
- Blinco , James P, et al. “Spin-Coated Carbon." Chemical Science, no. 9, 2013, pp. 3411–3415., doi:10.1039/C3SC51396C.
- Zheng, H., et al. “Effect of Sub 1-Nm Pt Nanoparticle on the Conduction Properties of Graphene Based Field Effect Transistor.” ECS Transactions, vol. 61, no. 39, Jan. 2014, pp. 1–11., doi:10.1149/06139.0001ecst.
- Mckitterick, Christopher B., et al. “Electron-Phonon cooling in large monolayer graphene devices.” Physical Review B, vol. 93, no. 7, Apr. 2016, doi:10.1103/physrevb.93.075410.
- Chan, Chun Yu. “Graphene based electrical biosensors for the detection of biomolecules.” The Hong Kong Polytechnic University, 2016.
- Pápa, Z., et al. “Spectroscopic ellipsometric investigation of graphene and thin carbon films from the point of view of depolarization effects.” Applied Surface Science, vol. 421, 2017, pp. 714–721., doi:10.1016/j.apsusc.2016.11.231.
- Hussain, Mushtaque, et al. “The improved piezoelectric properties of ZnO nanorods with oxygen plasma treatment on the single layer graphene coated polymer substrate.” Physica status solidi (a), vol. 211, no. 2, Oct. 2014, pp. 455–459., doi:10.1002/pssa.201300330.
- Kafiah, Feras, et al. “Synthesis of Graphene Based Membranes: Effect of Substrate Surface Properties on Monolayer Graphene Transfer.” Materials, vol. 10, no. 1, 2017, p. 86., doi:10.3390/ma10010086.
- Liang, Ji, et al. “Modulation of acousto-Electric current using a hybrid on-Chip AlN SAW/GFET device.” Applied Physics Letters, vol. 110, no. 24, Dec. 2017, p. 243504., doi:10.1063/1.4986481.
- Zhang, Yingjie & Kim, Youngseok & J. Gilbert, Matthew & Mason, Nadya. (2017). Electron transport in strain superlattices of graphene.
- Yan, Xiao-Qing, et al. “Polarization dependence of graphene transient optical response: interplay between incident direction and anisotropic distribution of nonequilibrium carriers.” Journal of the Optical Society of America B, vol. 34, no. 1, 2016, p. 218., doi:10.1364/josab.34.000218.
- Huang, Kun, et al. “Graphene coupled with Pt cubic nanoparticles for high performance, air-Stable graphene-Silicon solar cells.” Nano Energy, vol. 32, 2017, pp. 225–231., doi:10.1016/j.nanoen.2016.12.042.
- Lu, Bingyu, et al. “Roughened cylindrical gold layer with curve graphene coating for enhanced sensitivity of fiber SPR sensor.” 2017 19th International Conference on Solid-State Sensors, Actuators and Microsystems (TRANSDUCERS), 2017, doi:10.1109/transducers.2017.7994461.
- Kafiah, Feras, et al. “Synthesis of Graphene Based Membranes: Effect of Substrate Surface Properties on Monolayer Graphene Transfer.” Materials, vol. 10, no. 1, 2017, p. 86., doi:10.3390/ma10010086.
- Hsu, Wei-Hao, et al. “Low-Energy electron point projection microscopy/Diffraction study of suspended graphene.” Applied Surface Science, vol. 423, 30 Nov. 2017, pp. 266–274., doi:10.1016/j.apsusc.2017.06.148.
- Liang, Ji, et al. “Modulation of acousto-Electric current using a hybrid on-Chip AlN SAW/GFET device.” Applied Physics Letters, vol. 110, no. 24, Dec. 2017, p. 243504., doi:10.1063/1.4986481.
- Peng, Shuhua, et al. “Microwetting of Supported Graphene on Hydrophobic Surfaces Revealed by Polymerized Interfacial Femtodroplets.” Langmuir, vol. 30, no. 33, 2014, pp. 10043–10049., doi:10.1021/la5022774.
- Horvath, Cameron, et al. “Edge-Conformed silicon-Graphene waveguides: Fabrication and measurements.” 11th International Conference on Group IV Photonics (GFP), 2014, doi:10.1109/group4.2014.6961953.
- Watanabe, Hiroshi, et al. “Femtosecond midi-Infrared luminescence with hot-Phonon effects in graphenes and graphite.”
- Hassan, Saad. Transparent conductive article.
- Liang, Ji, et al. “Manipulation of carriers in graphene using an on-Chip acoustic wave device.” 2017 IEEE International Ultrasonics Symposium (IUS), 2017, doi:10.1109/ultsym.2017.8092898.
- Luo, Wen, et al. “Interaction of bimetallic PtCo layers with bare and graphene-Covered ZnO(0001) supports.” Surface Science, vol. 669, 2018, pp. 64–70., doi:10.1016/j.susc.2017.11.001.
- Jiang, Wen-Shuai, et al. “Preparation of high-Quality graphene using triggered microwave reduction under an air atmosphere.” Journal of Materials Chemistry C, 2018, doi:10.1039/c7tc03957c.
- Dong, Nannan, et al. “Pressure and Temperature Sensor Based on Graphene Diaphragm and Fiber Bragg Gratings.” IEEE Photonics Technology Letters, 2017, pp. 1–1., doi:10.1109/lpt.2017.2786292.
- Huczko, Andrzej, et al. “Efficient one-Pot combustion synthesis of few-Layered graphene.” Physica status solidi (b), vol. 252, no. 11, 2015, pp. 2412–2417., doi:10.1002/pssb.201552233.
- Lu, Bingyu, et al. “Roughened cylindrical gold layer with curve graphene coating for enhanced sensitivity of fiber SPR sensor.” 2017 19th International Conference on Solid-State Sensors, Actuators and Microsystems (TRANSDUCERS), 2017, doi:10.1109/transducers.2017.7994461.
- Fitri, Meika Aidil, et al. “Fabrication of TiO 2 -Graphene photocatalyst by direct chemical vapor deposition and its anti-Fouling property.” Materials Chemistry and Physics, vol. 198, 2017, pp. 42–48., doi:10.1016/j.matchemphys.2017.05.053.
- Bezryadin, A, et al. “Large energy storage efficiency of the dielectric layer of graphene nanocapacitors.” Nanotechnology, vol. 28, no. 49, 2017, p. 495401., doi:10.1088/1361-6528/aa935c.
- Shi, Cheng, et al. “Metamaterial-Based graphene thermal emitter.” Nano Research, June 2017, doi:10.1007/s12274-017-1922-7.
- McCaffrey, Debra L., et al. “Mechanism of ion adsorption to aqueous interfaces: Graphene/Water vs. air/Water.” Proceedings of the National Academy of Sciences, vol. 114, no. 51, 2017, pp. 13369–13373., doi:10.1073/pnas.1702760114.
- Zhang, Qin, et al. “Negative differential resistance and hysteresis in graphene-Based organic light-Emitting devices.” Journal of Materials Chemistry C, The Royal Society of Chemistry, 1 Jan. 2018, pubs.rsc.org/en/Content/ArticleLanding/2017/TC/C7TC05148D#!
- Miskin, Marc Z., et al. “Graphene-Based bimorphs for micron-Sized, autonomous origami machines.” Proceedings of the National Academy of Sciences, vol. 115, no. 3, Feb. 2018, pp. 466–470., doi:10.1073/pnas.1712889115.
- Bukola, Saheed, et al. “Selective Proton/Deuteron Transport through Nafion|Graphene|Nafion Sandwich Structures at High Current Density.” Journal of the American Chemical Society, vol. 140, no. 5, 2018, pp. 1743–1752., doi:10.1021/jacs.7b10853.
- Brown, Morgan A., et al. “Graphene Biotransistor Interfaced with a Nitrifying Biofilm.” Environmental Science & Technology Letters, vol. 2, no. 4, Mar. 2015, pp. 118–122., doi:10.1021/acs.estlett.5b00025.
- Woo, Sung Oh, and Winfried Teizer. “The effect of electron induced hydrogenation of graphene on its electrical transport properties.” Applied Physics Letters, vol. 103, no. 4, 2013, p. 041603., doi:10.1063/1.4816475.
- Li, Cheng, et al. “Manipulation of Nonlinear Optical Properties of Graphene Bonded Fiber Devices by Thermally Engineering Fermi-Dirac Distribution.” Advanced Optical Materials, vol. 5, no. 21, 2017, doi:10.1002/adom.201770103.
- Lock, Evgeniya H., et al. Stable IR Transparent Conductive Graphene Hybrid Materials and Methods of Making.
- Beechem, Thomas E., et al. “Self-Heating and Failure in Scalable Graphene Devices.” Scientific Reports, vol. 6, no. 1, Sept. 2016, doi:10.1038/srep26457.
- Ma, Yufeng, and Anni Siitonen. Chemical sensor using molecularly-Imprinted single layer graphene.
- Morrow, Wayne K., et al. “Role of graphene interlayers in mitigating degradation of Ni/Au ohmic contact morphology on p-Type GaN.” Vacuum, vol. 128, 2016, pp. 34–38., doi:10.1016/j.vacuum.2016.03.004.
- Nagamanasa, Kandula Hima, et al. “Liquid-Cell Electron Microscopy of Adsorbed Polymers.” Advanced Materials, vol. 29, no. 41, 2017, p. 1703555., doi:10.1002/adma.201703555.
- Hui, Fei. “Variability of graphene devices fabricated using graphene inks: Atomic force microscope tips.” Surface and Coatings Technology, vol. 320, 25 June 2017, doi:10.1016/j.surfcoat.2016.12.020.
- Chirayath, V A, et al. “Investigation of graphene using low energy positron annihilation induced Doppler broadening spectroscopy.” Journal of Physics: Conference Series, vol. 791, 2017, p. 012032., doi:10.1088/1742-6596/791/1/012032.
- Niu, Tianxiao, et al. “Indentation behavior of the stiffest membrane mounted on a very compliant substrate: Graphene on PDMS.” International Journal of Solids and Structures, vol. 132-133, 2018, pp. 1–8., doi:10.1016/j.ijsolstr.2017.05.038.
- Nashed, Ramy, et al. “Ultra-High Mobility in Dielectrically Pinned CVD Graphene.” IEEE Journal of the Electron Devices Society, vol. 4, no. 6, 2016, pp. 466–472., doi:10.1109/jeds.2016.2595498.
- Niu, Tianxiao, et al. “Fracture behavior of graphene mounted on stretchable substrate.” Carbon, vol. 109, 2016, pp. 852–859., doi:10.1016/j.carbon.2016.08.087.
- Boutilier, Michael S H, et al. “Knudsen effusion through polymer-Coated three-Layer porous graphene membranes.” Nanotechnology, vol. 28, no. 18, Oct. 2017, p. 184003., doi:10.1088/1361-6528/aa680f.
- Yokaribas, Volkan, et al. “Strain Gauges Based on CVD Graphene Layers and Exfoliated Graphene Nanoplatelets with Enhanced Reproducibility and Scalability for Large Quantities.” Sensors, vol. 17, no. 12, 2017, p. 2937., doi:10.3390/s17122937.
- Kuru, Cihan, et al. “Enhanced Power Conversion Efficiency of Graphene/Silicon Heterojunction Solar Cells Through NiO Induced Doping.” Journal of Nanoscience and Nanotechnology, vol. 16, no. 1, Jan. 2016, pp. 1190–1193., doi:10.1166/jnn.2016.12079.
|
-
FAQ
1.) Would you have any detail about the chemical composition of the silicon substrate itself? It's purity and % of oxygen within it for instance?
The Silicon wafers are doped with boron, with a doping concentration of 8*10^18/cm3; graphene on silicon wafers or silicon oxide wafers is insensitive to water and oxygen, and only graphene on copper substrates is sensitive to water and oxygen.
2.) Could you please let me know the mobility of The CVD Graphene on SiO2 Substrate (SKU#CVSO1P4D)?
The mobility of the CVD Graphene on SiO2 Substrate (SKU#CVSO1P4D) is ~1500.
3.) What is the grain size of graphene of this product?
CVD Graphene on SiO2 Substrate has a grain size of about 50 microns.
4.) Is the SiO2 on the backside of the substrate a natural oxide film?
It is not a natural oxide as the SiO2 is grown on the substrate. In addition, the backside SiO2 is also the same size as the topside. Please kindly refer to the SiO2 film thickness.
5.) Is the graphene on Silicon have no native Oxide?
Our product does not go through oxidation but it does have native Oxide (which is a tiny amount), however, if needed, we are able to customize it by removing the native Oxide.
Research Citations of ACS Material Products
- Chen, Ruiyi, et al. “Co-Percolating Graphene-Wrapped Silver Nanowire Network for High Performance, Highly Stable, Transparent Conducting Electrodes.” Advanced Functional Materials, vol. 23, no. 41, 2013, pp. 5150–5158., doi:10.1002/adfm.201300124.
- O’Hern, Sean C., et al. “Selective Molecular Transport through Intrinsic Defects in a Single Layer of CVD Graphene.” ACS Nano, vol. 6, no. 11, Sept. 2012, pp. 10130–10138., doi:10.1021/nn303869m.
- Yoo, Jae-Hyuck, et al. “Graphene folds by femtosecond laser ablation.” Applied Physics Letters, vol. 100, no. 23, Apr. 2012, p. 233124., doi:10.1063/1.4724213.
- Longchamp, Jean-Nicolas, et al. “Low-Energy electron transmission imaging of clusters on free-Standing graphene.” Applied Physics Letters, vol. 101, no. 11, Oct. 2012, p. 113117., doi:10.1063/1.4752717.
- Chen, Xu-Dong, et al. “High-Quality and efficient transfer of large-Area graphene films onto different substrates.” Carbon, vol. 56, May 2013, pp. 271–278., doi:10.1016/j.carbon.2013.01.011.
- Ye, Qing., et al. “Polarization-Dependent optical absorption of graphene under total internal reflection.” Applied Physics Letters, vol. 102, no. 2, doi:10.1063/1.4776694.
- Longchamp, Jean-Nicolas, et al. “Ultraclean freestanding graphene by platinum-Metal catalysis.” Journal of Vacuum Science & Technology B, Nanotechnology and Microelectronics: Materials, Processing, Measurement, and Phenomena, vol. 31, no. 2, 2013, p. 020605., doi:10.1116/1.4793746.
- Wang, Yung Yu, and Peter J. Burke. “A large-Area and contamination-Free graphene transistor for liquid-Gated sensing applications.” Applied Physics Letters, vol. 103, no. 5, 2013, p. 052103., doi:10.1063/1.4816764.
- Wang, Peng, et al. “Accurate layers determination of graphene on transparent substrate based on polarization-Sensitive absorption effect.” Applied Physics Letters, vol. 103, no. 18, 2013, p. 181902., doi:10.1063/1.4827812.
- Zhou, Rong, et al. “Large-Energy, narrow-Bandwidth laser pulse at 1645 nm in a diode-Pumped Er:YAG solid-State laser passively Q-Switched by a monolayer graphene saturable absorber.” Applied Optics, vol. 53, no. 2, Sept. 2014, p. 254., doi:10.1364/ao.53.000254.
- Choi, Duyoung, et al. “Nanopatterned Graphene Field Effect Transistor Fabricated Using Block Co-Polymer Lithography.” Materials Research Letters, vol. 2, no. 3, Sept. 2014, pp. 131–139., doi:10.1080/21663831.2013.876676.
- Srisonphan, Siwapon, et al. “Space charge neutralization by electron-Transparent suspended graphene.” Scientific Reports, vol. 4, no. 1, 2014, doi:10.1038/srep03764.
- Joiner, C. A., et al. “Cleaning graphene with a titanium sacrificial layer.” Applied Physics Letters, vol. 104, no. 22, Feb. 2014, p. 223109., doi:10.1063/1.4881886.
- Roy, T., et al. “Tunneling characteristics in chemical vapor deposited graphene–hexagonal boron nitride–graphene junctions.” Applied Physics Letters, vol. 104, no. 12, 2014, p. 123506., doi:10.1063/1.4870073.
- Burke, Peter J. “Charging the Quantum Capacitance of Graphene with a Single Biological Ion Channel.” Biophysical Journal, vol. 106, no. 2, 2014, doi:10.1016/j.bpj.2013.11.2796.
- Li, Peining, et al. “Graphene-Enhanced Infrared Near-Field Microscopy.” Nano Letters, vol. 14, no. 8, 2014, pp. 4400–4405., doi:10.1021/nl501376a.
- Boutilier, Michael S. H., et al. “Implications of Permeation through Intrinsic Defects in Graphene on the Design of Defect-Tolerant Membranes for Gas Separation.” ACS Nano, vol. 8, no. 1, Mar. 2014, pp. 841–849., doi:10.1021/nn405537u.
- Lee, Jiye, et al. “Switching Individual Quantum Dot Emission through Electrically Controlling Resonant Energy Transfer to Graphene.” Nano Letters, vol. 14, no. 12, 2014, pp. 7115–7119., doi:10.1021/nl503587z.
- Srisonphan, Siwapon, and Komsan Hongesombut. “Tuning the ballistic electron transport of spatial graphene–metal sandwich electrode on a vacuum-Silicon-Based device.” RSC Advances, vol. 5, no. 3, 2015, pp. 2032–2037., doi:10.1039/c4ra09503k.
- Hui, Fei, et al. “Mechanical properties of locally oxidized graphene electrodes.” Archive of Applied Mechanics, vol. 85, no. 3, 2014, pp. 339–345., doi:10.1007/s00419-014-0957-4.
- Fujimoto, A, et al. “Negative magnetoresistance in Ti-Cleaned single-Layer graphene.” Journal of Physics: Conference Series, vol. 603, 2015, p. 012021., doi:10.1088/1742-6596/603/1/012021.
- Thareja, Vrinda, et al. “Electrically Tunable Coherent Optical Absorption in Graphene with Ion Gel.” Nano Letters, vol. 15, no. 3, 2015, pp. 1570–1576., doi:10.1021/nl503431d.
- Ye, X. H., et al. “Corrosion resistance of graphene directly and locally grown on bulk nickel substrate by laser irradiation.” RSC Advances, vol. 5, no. 45, 2015, pp. 35384–35390., doi:10.1039/c5ra01267h.
- Horvath, Cameron . “Fabrication and Characterization of Edge-Conformed Graphene-Silicon Waveguides.” IEEE Photonics Technology Letters, vol. 27, no. 6, pp. 585–587., doi:10.1109/LPT.2014.2385757.
- Marta, Bogdan, et al. “Efficient etching-Free transfer of high quality, large-Area CVD grown graphene onto polyvinyl alcohol films.” Applied Surface Science, vol. 363, 2016, pp. 613–618., doi:10.1016/j.apsusc.2015.11.265.
- Zheng, Guanpeng, et al. “Improved Transfer Quality of CVD-Grown Graphene by Ultrasonic Processing of Target Substrates: Applications for Ultra-Fast Laser Photonics.” ACS Applied Materials & Interfaces, vol. 5, no. 20, Sept. 2013, pp. 10288–10293., doi:10.1021/am403205v.
- Zeng, Yong, et al. “Investigate the interface structure and growth mechanism of high quality ZnO films grown on multilayer graphene layers.” Applied Surface Science, vol. 301, 2014, pp. 391–395., doi:10.1016/j.apsusc.2014.02.088.
- Ye, Qing, et al. “Polarization-Dependent optical absorption of graphene under total internal reflection.” Applied Physics Letters, vol. 102, no. 2, Jan. 2013, doi:10.1063/1.4776694.
- Watanabe, Hiroshi, et al. “Layer number dependence of carrier lifetime in graphenes observed using time-Resolved mid-Infrared luminescence.” Chemical Physics Letters, vol. 637, 2015, pp. 58–62., doi:10.1016/j.cplett.2015.07.046.
- He, Yingbo, et al. “Strongly enhanced Raman scattering of graphene by a single gold nanorod.” Applied Physics Letters, vol. 107, no. 5, Mar. 2015, p. 053104., doi:10.1063/1.4927759.
- Choi, Duyoung, et al. “Uniformly Nanopatterned Graphene Field-Effect Transistors with Enhanced Properties.” Nanoscale Research Letters, vol. 10, no. 1, Nov. 2015, doi:10.1186/s11671-015-0976-2.
- Miao, Lili, et al. “Broadband ultrafast nonlinear optical response of few-Layers graphene: toward the mid-Infrared regime.” Photonics Research, vol. 3, no. 5, 2015, p. 214., doi:10.1364/prj.3.000214.
- Hu, Jianchen, et al. “Improvement of the electrical contact resistance at rough interfaces using two dimensional materials.” Journal of Applied Physics, vol. 118, no. 21, July 2015, p. 215301., doi:10.1063/1.4936366.
- Jain, Tarun, et al. “Heterogeneous sub-Continuum ionic transport in statistically isolated graphene nanopores.” Nature Nanotechnology, vol. 10, no. 12, May 2015, pp. 1053–1057., doi:10.1038/nnano.2015.222.
- Srisonphan, Siwapon, and Komsan Hongesombut. “Tuning the ballistic electron transport of spatial graphene–metal sandwich electrode on a vacuum-Silicon-Based device.” RSC Advances, vol. 5, no. 3, 2015, pp. 2032–2037., doi:10.1039/c4ra09503k.
- Liu, Xiangjiang, et al. “Compact Shielding of Graphene Monolayer Leads to Extraordinary SERS-Active Substrate with Large-Area Uniformity and Long-Term Stability.” Scientific Reports, vol. 5, no. 1, 2015, doi:10.1038/srep17167.
- Hui, Fei, et al. “Moving graphene devices from lab to market: advanced graphene-Coated nanoprobes.” Nanoscale, vol. 8, no. 16, 2016, pp. 8466–8473., doi:10.1039/c5nr06235g.
- Ye, Xiaohui, et al. “Protecting carbon steel from corrosion by laser in situ grown graphene films.” Carbon, vol. 94, 2015, pp. 326–334., doi:10.1016/j.carbon.2015.06.080.
- Wu, Man, et al. “Wavelength switchable graphene Q-Switched fiber laser with cascaded fiber Bragg gratings.” Optics Communications, vol. 368, 2016, pp. 81–85., doi:10.1016/j.optcom.2016.01.069.
- Mackin, Charles, and Tomás Palacios. “Large-Scale sensor systems based on graphene electrolyte-Gated field-Effect transistors.” The Analyst, vol. 141, no. 9, 2016, pp. 2704–2711., doi:10.1039/c5an02328a.
- Politou, Maria, et al. “Multi-Layer graphene interconnect.” 2016 IEEE International Interconnect Technology Conference / Advanced Metallization Conference (IITC/AMC), July 2016, doi:10.1109/IITC-AMC.2016.7507731.
- Cao, Zhengmin, et al. “Nano-Gap between a gold tip and nanorod for polarization dependent surface enhanced Raman scattering.” Applied Physics Letters, vol. 109, no. 23, May 2016, p. 233103., doi:10.1063/1.4971832.
- Deng, Xiangquan, et al. “Terahertz-Induced photothermoelectric response in graphene-Metal contact structures.” Journal of Physics D: Applied Physics, vol. 49, no. 42, 2016, p. 425101., doi:10.1088/0022-3727/49/42/425101.
- Guo, Chu-Cai, et al. “Experimental Demonstration of Total Absorption over 99% in the Near Infrared for Monolayer-Graphene-Based Subwavelength Structures.” Advanced Optical Materials, vol. 4, no. 12, Jan. 2016, pp. 1955–1960., doi:10.1002/adom.201600481.
- Hu, Jianbo, et al. “Rippling ultrafast dynamics of suspended 2D monolayers, graphene.” Proceedings of the National Academy of Sciences, vol. 113, no. 43, Oct. 2016, doi:10.1073/pnas.1613818113.
- Li, Cheng, et al. “Measurement of the Adhesion Energy of Pressurized Graphene Diaphragm Using Optical Fiber Fabry–Perot Interference.” IEEE Sensors Journal, vol. 16, no. 10, 2016, pp. 3664–3669., doi:10.1109/jsen.2016.2536783.
- Yuan, Jun, et al. “Modulation of far-Infrared light transmission by graphene-Silicon Schottky junction.” Optical Materials Express, vol. 6, no. 12, 2016, p. 3908., doi:10.1364/ome.6.003908.
- Li, Cheng, et al. “Measurement of thermal expansion coefficient of graphene diaphragm using optical fiber Fabry–Perot interference.” Measurement Science and Technology, vol. 27, no. 7, 2016, p. 075102., doi:10.1088/0957-0233/27/7/075102.
- Kafiah, Feras M., et al. “Monolayer graphene transfer onto polypropylene and polyvinylidenedifluoride microfiltration membranes for water desalination.” Desalination, vol. 388, 2016, pp. 29–37., doi:10.1016/j.desal.2016.02.027.
- Wu, Xiangyu, et al. “Doping of graphene for the application in nano-Interconnect.” Microelectronic Engineering, vol. 167, 5 Jan. 2017, pp. 42–46., doi:10.1016/j.mee.2016.10.013.
- Liu, Siyang, et al. “Atomic emission spectroscopy of electrically triggered exploding nanoparticle analytes on graphene/SiO2/Si substrate.” Journal of Vacuum Science & Technology B, Nanotechnology and Microelectronics: Materials, Processing, Measurement, and Phenomena, vol. 34, no. 6, 2016, doi:10.1116/1.4964819.
- Sattler, Klaus D. Carbon nanomaterials sourcebook. Vol. 1, CRC Press, 2016.
- Politou, Maria, et al. “Evaluation of multilayer graphene for advanced interconnects.” Microelectronic Engineering, vol. 167, 5 Jan. 2017, pp. 1–5., doi:10.1016/j.mee.2016.09.011.
- Hwang, Michael T., et al. “Highly specific SNP detection using 2D graphene electronics and DNA strand displacement.” Proceedings of the National Academy of Sciences, vol. 113, no. 26, 2016, pp. 7088–7093., doi:10.1073/pnas.1603753113.
- Nieto, Andy. “Graphene reinforced metal and ceramic matrix composites: a review.” International Materials Reviews, vol. 62, no. 5, 27 Oct. 2017, pp. 241–302., doi:10.1080/09506608.2016.1219481.
- Khorasaninejad, M., et al. “Highly Enhanced Raman Scattering of Graphene using Plasmonic Nano-Structure.” Scientific Reports, vol. 3, no. 1, 2013, doi:10.1038/srep02936.
- Kunz, Daniel A., et al. “Space-Resolved In-Plane Moduli of Graphene Oxide and Chemically Derived Graphene Applying a Simple Wrinkling Procedure (Adv. Mater. 9/2013).” Advanced Materials, vol. 25, no. 9, Apr. 2013, pp. 1336–1336., doi:10.1002/adma.201370060.
- Peng, Shuhua, et al. “Microwetting of Supported Graphene on Hydrophobic Surfaces Revealed by Polymerized Interfacial Femtodroplets.” Langmuir, vol. 30, no. 33, 2014, pp. 10043–10049., doi:10.1021/la5022774.
- Luo, Wen, et al. “Single-Layer Graphene as an Effective Mediator of the Metal–Support Interaction.” The Journal of Physical Chemistry Letters, vol. 5, no. 11, 2014, pp. 1837–1844., doi:10.1021/jz500425j.
- Suemoto, Tohru, et al. “Thickness dependent hot-Phonon effects observed by femtosecond mid-Infrared luminescence in graphene.” 19th International Conference on Ultrafast Phenomena, 2014, doi:10.1364/up.2014.07.mon.p1.32.
- Zhou, Haosen, et al. “Influence of CO2 On the Stability of Discharge Performance for Li-Air Battery with Hybrid Electrolyte Based On the Graphene Sheet.” Conference: 224th ECS Meeting. Oct. 2013, doi:10.1039/c3ra47258b.
- Thareja, Vrinda. “Electrically Tunable Optical Absorption in a Graphene-Based Salisbury Screen.” CORNELL UNIVERSITY LIBRARY, 7 Sept. 2014.
- Horvath, Cameron S. “Light Propagation and Photothermal Nonlinearity in Graphene-Si Waveguides .” University of Alberta Libraries , Nov. 2013, doi:10.7939/R3ST1N.
- Li, Cheng, et al. “Interference characteristics in a Fabry–Perot cavity with graphene membrane for optical fiber pressure sensors.” Microsystem Technologies, vol. 21, no. 11, 2014, pp. 2297–2306., doi:10.1007/s00542-014-2333-2.
- Kou, Jun-Long, et al. “Platform for enhanced light–graphene interaction length and miniaturizing fiber stereo devices.” Optica, vol. 1, no. 5, 2014, p. 307., doi:10.1364/optica.1.000307.
- Karnik, Rohit. “Ionic and Molecular Transport Through Graphene Membranes.” Transport and Reactivity of Solutions in Confined Hydrosystems NATO Science for Peace and Security Series C: Environmental Security, Dec. 2013, pp. 95–102., doi:10.1007/978-94-007-7534-3_8.
- Warren, A. J., et al. “In vacuo X-Ray data collection from graphene-Wrapped protein crystals.” Acta Crystallographica Section D STRUCTURAL BIOLOGY, vol. D71, 2015, pp. 2079–2088., doi:10.1107/S1399004715014194.
- Wen, Chenyu, et al. “Assessing kinetics of surface adsorption–desorption of gas molecules via electrical measurements.” Sensors and Actuators B: Chemical, vol. 223, 2016, pp. 791–798., doi:10.1016/j.snb.2015.10.019.
- Li, Cheng, et al. “Analyzing the applicability of miniature ultra-High sensitivity Fabry–Perot acoustic sensor using a nanothick graphene diaphragm.” Measurement Science and Technology, vol. 26, no. 8, 10 July 2015.
- Li, Cheng, et al. “Analyzing the temperature sensitivity of Fabry-Perot sensor using multilayer graphene diaphragm.” OSA Publishing, vol. 23, no. 21, 2015, pp. 27494–27502., doi:10.1364/OE.23.027494.
- Lau, K. Y., et al. “High Signal-To-Noise Ratio Q-Switching Erbium Doped Fiber Laser Pulse Emission Utilizing Single Layer Trivial Transfer Graphene Film Saturable Absorber.” Jurnal Teknologi, vol. 78, no. 3, 2016, doi:10.11113/jt.v78.7478.
- Zheng, Shijun, et al. “Acoustic charge transport induced by the surface acoustic wave in chemical doped graphene.” Applied Physics Letters, vol. 109, no. 18, 2016, p. 183110., doi:10.1063/1.4967192.
- Deng, Xiangquan, et al. “Terahertz-Induced photothermoelectric response in graphene-Metal contact structures.” Journal of Physics D: Applied Physics, vol. 49, no. 42, 2016, p. 425101., doi:10.1088/0022-3727/49/42/425101.
- Luo, Wen, and Spyridon Zafeiratos. “Inside Back Cover: Graphene-Coated ZnO and SiO2 as Supports for CoO Nanoparticles with Enhanced Reducibility.” ChemPhysChem, vol. 17, no. 19, Apr. 2016, pp. 3146–3146., doi:10.1002/cphc.201601023.
- Lau, K.Y., et al. “Passively mode-Locked soliton femtosecond pulses employing graphene saturable absorber.” Optics & Laser Technology, vol. 94, 2017, pp. 221–227., doi:10.1016/j.optlastec.2017.03.035.
- Fitri, Meika Aidil, et al. “Fabrication of TiO 2 -Graphene photocatalyst by direct chemical vapor deposition and its anti-Fouling property.” Materials Chemistry and Physics, vol. 198, 2017, pp. 42–48., doi:10.1016/j.matchemphys.2017.05.053.
- Li, Cheng, et al. “Nondestructive andin situdetermination of graphene layers using optical fiber Fabry–Perot interference.” Measurement Science and Technology, vol. 28, no. 2, Dec. 2017, p. 025206., doi:10.1088/1361-6501/aa54f8.
- Gao, Xiangyang, et al. “Measuring Graphene Adhesion on Silicon Substrate by Single and Dual Nanoparticle-Loaded Blister.” Advanced Materials Interfaces, vol. 4, no. 9, 2017, p. 1601023., doi:10.1002/admi.201601023.
- Tang, Xiaoduan, et al. “Five Orders of Magnitude Reduction in Energy Coupling across Corrugated Graphene/Substrate Interfaces.” ACS Applied Materials & Interfaces, vol. 6, no. 4, July 2014, pp. 2809–2818., doi:10.1021/am405388a.
- Morrow, W. K., et al. “(Invited) The Use of Graphene as a Solid State Diffusion Barrier.” ECS Transactions, vol. 61, no. 4, 2014, pp. 371–379., doi:10.1149/06104.0371ecst.
- Zheng, Haisheng, et al. “Ultrafine Pt nanoparticle induced doping/Strain of single layer graphene: experimental corroboration between conduction and Raman characteristics.” Journal of Materials Science: Materials in Electronics, vol. 26, no. 7, 2015, pp. 4746–4753., doi:10.1007/s10854-015-3043-y.
- Blinco , James P, et al. “Spin-Coated Carbon." Chemical Science, no. 9, 2013, pp. 3411–3415., doi:10.1039/C3SC51396C.
- Zheng, H., et al. “Effect of Sub 1-Nm Pt Nanoparticle on the Conduction Properties of Graphene Based Field Effect Transistor.” ECS Transactions, vol. 61, no. 39, Jan. 2014, pp. 1–11., doi:10.1149/06139.0001ecst.
- Mckitterick, Christopher B., et al. “Electron-Phonon cooling in large monolayer graphene devices.” Physical Review B, vol. 93, no. 7, Apr. 2016, doi:10.1103/physrevb.93.075410.
- Chan, Chun Yu. “Graphene based electrical biosensors for the detection of biomolecules.” The Hong Kong Polytechnic University, 2016.
- Pápa, Z., et al. “Spectroscopic ellipsometric investigation of graphene and thin carbon films from the point of view of depolarization effects.” Applied Surface Science, vol. 421, 2017, pp. 714–721., doi:10.1016/j.apsusc.2016.11.231.
- Hussain, Mushtaque, et al. “The improved piezoelectric properties of ZnO nanorods with oxygen plasma treatment on the single layer graphene coated polymer substrate.” Physica status solidi (a), vol. 211, no. 2, Oct. 2014, pp. 455–459., doi:10.1002/pssa.201300330.
- Kafiah, Feras, et al. “Synthesis of Graphene Based Membranes: Effect of Substrate Surface Properties on Monolayer Graphene Transfer.” Materials, vol. 10, no. 1, 2017, p. 86., doi:10.3390/ma10010086.
- Liang, Ji, et al. “Modulation of acousto-Electric current using a hybrid on-Chip AlN SAW/GFET device.” Applied Physics Letters, vol. 110, no. 24, Dec. 2017, p. 243504., doi:10.1063/1.4986481.
- Zhang, Yingjie & Kim, Youngseok & J. Gilbert, Matthew & Mason, Nadya. (2017). Electron transport in strain superlattices of graphene.
- Yan, Xiao-Qing, et al. “Polarization dependence of graphene transient optical response: interplay between incident direction and anisotropic distribution of nonequilibrium carriers.” Journal of the Optical Society of America B, vol. 34, no. 1, 2016, p. 218., doi:10.1364/josab.34.000218.
- Huang, Kun, et al. “Graphene coupled with Pt cubic nanoparticles for high performance, air-Stable graphene-Silicon solar cells.” Nano Energy, vol. 32, 2017, pp. 225–231., doi:10.1016/j.nanoen.2016.12.042.
- Lu, Bingyu, et al. “Roughened cylindrical gold layer with curve graphene coating for enhanced sensitivity of fiber SPR sensor.” 2017 19th International Conference on Solid-State Sensors, Actuators and Microsystems (TRANSDUCERS), 2017, doi:10.1109/transducers.2017.7994461.
- Kafiah, Feras, et al. “Synthesis of Graphene Based Membranes: Effect of Substrate Surface Properties on Monolayer Graphene Transfer.” Materials, vol. 10, no. 1, 2017, p. 86., doi:10.3390/ma10010086.
- Hsu, Wei-Hao, et al. “Low-Energy electron point projection microscopy/Diffraction study of suspended graphene.” Applied Surface Science, vol. 423, 30 Nov. 2017, pp. 266–274., doi:10.1016/j.apsusc.2017.06.148.
- Peng, Shuhua, et al. “Microwetting of Supported Graphene on Hydrophobic Surfaces Revealed by Polymerized Interfacial Femtodroplets.” Langmuir, vol. 30, no. 33, 2014, pp. 10043–10049., doi:10.1021/la5022774.
- Horvath, Cameron, et al. “Edge-Conformed silicon-Graphene waveguides: Fabrication and measurements.” 11th International Conference on Group IV Photonics (GFP), 2014, doi:10.1109/group4.2014.6961953.
- Watanabe, Hiroshi, et al. “Femtosecond midi-Infrared luminescence with hot-Phonon effects in graphenes and graphite.”
- Hassan, Saad. Transparent conductive article.
- Liang, Ji, et al. “Manipulation of carriers in graphene using an on-Chip acoustic wave device.” 2017 IEEE International Ultrasonics Symposium (IUS), 2017, doi:10.1109/ultsym.2017.8092898.
- Luo, Wen, et al. “Interaction of bimetallic PtCo layers with bare and graphene-Covered ZnO(0001) supports.” Surface Science, vol. 669, 2018, pp. 64–70., doi:10.1016/j.susc.2017.11.001.
- Jiang, Wen-Shuai, et al. “Preparation of high-Quality graphene using triggered microwave reduction under an air atmosphere.” Journal of Materials Chemistry C, 2018, doi:10.1039/c7tc03957c.
- Dong, Nannan, et al. “Pressure and Temperature Sensor Based on Graphene Diaphragm and Fiber Bragg Gratings.” IEEE Photonics Technology Letters, 2017, pp. 1–1., doi:10.1109/lpt.2017.2786292.
- Huczko, Andrzej, et al. “Efficient one-Pot combustion synthesis of few-Layered graphene.” Physica status solidi (b), vol. 252, no. 11, 2015, pp. 2412–2417., doi:10.1002/pssb.201552233.
- Lu, Bingyu, et al. “Roughened cylindrical gold layer with curve graphene coating for enhanced sensitivity of fiber SPR sensor.” 2017 19th International Conference on Solid-State Sensors, Actuators and Microsystems (TRANSDUCERS), 2017, doi:10.1109/transducers.2017.7994461.
- Fitri, Meika Aidil, et al. “Fabrication of TiO 2 -Graphene photocatalyst by direct chemical vapor deposition and its anti-Fouling property.” Materials Chemistry and Physics, vol. 198, 2017, pp. 42–48., doi:10.1016/j.matchemphys.2017.05.053.
- Bezryadin, A, et al. “Large energy storage efficiency of the dielectric layer of graphene nanocapacitors.” Nanotechnology, vol. 28, no. 49, 2017, p. 495401., doi:10.1088/1361-6528/aa935c.
- Shi, Cheng, et al. “Metamaterial-Based graphene thermal emitter.” Nano Research, June 2017, doi:10.1007/s12274-017-1922-7.
- McCaffrey, Debra L., et al. “Mechanism of ion adsorption to aqueous interfaces: Graphene/Water vs. air/Water.” Proceedings of the National Academy of Sciences, vol. 114, no. 51, 2017, pp. 13369–13373., doi:10.1073/pnas.1702760114.
- Zhang, Qin, et al. “Negative differential resistance and hysteresis in graphene-Based organic light-Emitting devices.” Journal of Materials Chemistry C, The Royal Society of Chemistry, 1 Jan. 2018, pubs.rsc.org/en/Content/ArticleLanding/2017/TC/C7TC05148D#!
- Miskin, Marc Z., et al. “Graphene-Based bimorphs for micron-Sized, autonomous origami machines.” Proceedings of the National Academy of Sciences, vol. 115, no. 3, Feb. 2018, pp. 466–470., doi:10.1073/pnas.1712889115.
- Bukola, Saheed, et al. “Selective Proton/Deuteron Transport through Nafion|Graphene|Nafion Sandwich Structures at High Current Density.” Journal of the American Chemical Society, vol. 140, no. 5, 2018, pp. 1743–1752., doi:10.1021/jacs.7b10853.
- Brown, Morgan A., et al. “Graphene Biotransistor Interfaced with a Nitrifying Biofilm.” Environmental Science & Technology Letters, vol. 2, no. 4, Mar. 2015, pp. 118–122., doi:10.1021/acs.estlett.5b00025.
- Woo, Sung Oh, and Winfried Teizer. “The effect of electron induced hydrogenation of graphene on its electrical transport properties.” Applied Physics Letters, vol. 103, no. 4, 2013, p. 041603., doi:10.1063/1.4816475.
- Li, Cheng, et al. “Manipulation of Nonlinear Optical Properties of Graphene Bonded Fiber Devices by Thermally Engineering Fermi-Dirac Distribution.” Advanced Optical Materials, vol. 5, no. 21, 2017, doi:10.1002/adom.201770103.
- Chirayath, V. A, et al. “Positron induced electron emission from graphene.” IOP Science, Journal of Physics: Conference Series.
- Lock, Evgeniya H., et al. Stable IR Transparent Conductive Graphene Hybrid Materials and Methods of Making.
- Beechem, Thomas E., et al. “Self-Heating and Failure in Scalable Graphene Devices.” Scientific Reports, vol. 6, no. 1, Sept. 2016, doi:10.1038/srep26457.
- Ma, Yufeng, and Anni Siitonen. Chemical sensor using molecularly-Imprinted single layer graphene.
- Morrow, Wayne K., et al. “Role of graphene interlayers in mitigating degradation of Ni/Au ohmic contact morphology on p-Type GaN.” Vacuum, vol. 128, 2016, pp. 34–38., doi:10.1016/j.vacuum.2016.03.004.
- Nagamanasa, Kandula Hima, et al. “Liquid-Cell Electron Microscopy of Adsorbed Polymers.” Advanced Materials, vol. 29, no. 41, 2017, p. 1703555., doi:10.1002/adma.201703555.
- Hui, Fei. “Variability of graphene devices fabricated using graphene inks: Atomic force microscope tips.” Surface and Coatings Technology, vol. 320, 25 June 2017, doi:10.1016/j.surfcoat.2016.12.020.
- Chirayath, V A, et al. “Investigation of graphene using low energy positron annihilation induced Doppler broadening spectroscopy.” Journal of Physics: Conference Series, vol. 791, 2017, p. 012032., doi:10.1088/1742-6596/791/1/012032.
- Niu, Tianxiao, et al. “Indentation behavior of the stiffest membrane mounted on a very compliant substrate: Graphene on PDMS.” International Journal of Solids and Structures, vol. 132-133, 2018, pp. 1–8., doi:10.1016/j.ijsolstr.2017.05.038.
- Niu, Tianxiao, et al. “Fracture behavior of graphene mounted on stretchable substrate.” Carbon, vol. 109, 2016, pp. 852–859., doi:10.1016/j.carbon.2016.08.087.
- Boutilier, Michael S H, et al. “Knudsen effusion through polymer-Coated three-Layer porous graphene membranes.” Nanotechnology, vol. 28, no. 18, Oct. 2017, p. 184003., doi:10.1088/1361-6528/aa680f.
- Du, Feng, et al. “Surface stress of graphene layers supported on soft substrate.” Scientific Reports, vol. 6, no. 1, Nov. 2016, doi:10.1038/srep25653.
- Yokaribas, Volkan, et al. “Strain Gauges Based on CVD Graphene Layers and Exfoliated Graphene Nanoplatelets with Enhanced Reproducibility and Scalability for Large Quantities.” Sensors, vol. 17, no. 12, 2017, p. 2937., doi:10.3390/s17122937.
- Kuru, Cihan, et al. “Enhanced Power Conversion Efficiency of Graphene/Silicon Heterojunction Solar Cells Through NiO Induced Doping.” Journal of Nanoscience and Nanotechnology, vol. 16, no. 1, Jan. 2016, pp. 1190–1193., doi:10.1166/jnn.2016.12079.
- Politou, Maria. "Graphene-Metal Contact Engineering and Graphene Interconnects." (2019).
|
-
FAQ
1. Is the substrate doped? What's the type?
It is heavily doped p-type Si wafer and the electrical resistivity is about 0.01 to 0.02 Ω·cm.
2. What is the clear transparent sticky layer that holds the sample? What is its purpose?
The clear transparent sticky layer is a PET material. The only purpose of this layer is to hold the sample in its place and to ensure the sample remains intact during transportation.
Research Citations of ACS Material Products
- Chen, Ruiyi, et al. “Co-Percolating Graphene-Wrapped Silver Nanowire Network for High Performance, Highly Stable, Transparent Conducting Electrodes.” Advanced Functional Materials, vol. 23, no. 41, 2013, pp. 5150–5158., doi:10.1002/adfm.201300124.
- O’Hern, Sean C., et al. “Selective Molecular Transport through Intrinsic Defects in a Single Layer of CVD Graphene.” ACS Nano, vol. 6, no. 11, Sept. 2012, pp. 10130–10138., doi:10.1021/nn303869m.
- Yoo, Jae-Hyuck, et al. “Graphene folds by femtosecond laser ablation.” Applied Physics Letters, vol. 100, no. 23, Apr. 2012, p. 233124., doi:10.1063/1.4724213.
- Longchamp, Jean-Nicolas, et al. “Low-Energy electron transmission imaging of clusters on free-Standing graphene.” Applied Physics Letters, vol. 101, no. 11, Oct. 2012, p. 113117., doi:10.1063/1.4752717.
- Chen, Xu-Dong, et al. “High-Quality and efficient transfer of large-Area graphene films onto different substrates.” Carbon, vol. 56, May 2013, pp. 271–278., doi:10.1016/j.carbon.2013.01.011.
- Ye, Qing., et al. “Polarization-Dependent optical absorption of graphene under total internal reflection.” Applied Physics Letters, vol. 102, no. 2, doi:10.1063/1.4776694.
- Longchamp, Jean-Nicolas, et al. “Ultraclean freestanding graphene by platinum-Metal catalysis.” Journal of Vacuum Science & Technology B, Nanotechnology and Microelectronics: Materials, Processing, Measurement, and Phenomena, vol. 31, no. 2, 2013, p. 020605., doi:10.1116/1.4793746.
- Wang, Yung Yu, and Peter J. Burke. “A large-Area and contamination-Free graphene transistor for liquid-Gated sensing applications.” Applied Physics Letters, vol. 103, no. 5, 2013, p. 052103., doi:10.1063/1.4816764.
- Wang, Peng, et al. “Accurate layers determination of graphene on transparent substrate based on polarization-Sensitive absorption effect.” Applied Physics Letters, vol. 103, no. 18, 2013, p. 181902., doi:10.1063/1.4827812.
- Zhou, Rong, et al. “Large-Energy, narrow-Bandwidth laser pulse at 1645 nm in a diode-Pumped Er:YAG solid-State laser passively Q-Switched by a monolayer graphene saturable absorber.” Applied Optics, vol. 53, no. 2, Sept. 2014, p. 254., doi:10.1364/ao.53.000254.
- Choi, Duyoung, et al. “Nanopatterned Graphene Field Effect Transistor Fabricated Using Block Co-Polymer Lithography.” Materials Research Letters, vol. 2, no. 3, Sept. 2014, pp. 131–139., doi:10.1080/21663831.2013.876676.
- Srisonphan, Siwapon, et al. “Space charge neutralization by electron-Transparent suspended graphene.” Scientific Reports, vol. 4, no. 1, 2014, doi:10.1038/srep03764.
- Joiner, C. A., et al. “Cleaning graphene with a titanium sacrificial layer.” Applied Physics Letters, vol. 104, no. 22, Feb. 2014, p. 223109., doi:10.1063/1.4881886.
- Roy, T., et al. “Tunneling characteristics in chemical vapor deposited graphene–hexagonal boron nitride–graphene junctions.” Applied Physics Letters, vol. 104, no. 12, 2014, p. 123506., doi:10.1063/1.4870073.
- Burke, Peter J. “Charging the Quantum Capacitance of Graphene with a Single Biological Ion Channel.” Biophysical Journal, vol. 106, no. 2, 2014, doi:10.1016/j.bpj.2013.11.2796.
- Li, Peining, et al. “Graphene-Enhanced Infrared Near-Field Microscopy.” Nano Letters, vol. 14, no. 8, 2014, pp. 4400–4405., doi:10.1021/nl501376a.
- Boutilier, Michael S. H., et al. “Implications of Permeation through Intrinsic Defects in Graphene on the Design of Defect-Tolerant Membranes for Gas Separation.” ACS Nano, vol. 8, no. 1, Mar. 2014, pp. 841–849., doi:10.1021/nn405537u.
- Lee, Jiye, et al. “Switching Individual Quantum Dot Emission through Electrically Controlling Resonant Energy Transfer to Graphene.” Nano Letters, vol. 14, no. 12, 2014, pp. 7115–7119., doi:10.1021/nl503587z.
- Srisonphan, Siwapon, and Komsan Hongesombut. “Tuning the ballistic electron transport of spatial graphene–metal sandwich electrode on a vacuum-Silicon-Based device.” RSC Advances, vol. 5, no. 3, 2015, pp. 2032–2037., doi:10.1039/c4ra09503k.
- Hui, Fei, et al. “Mechanical properties of locally oxidized graphene electrodes.” Archive of Applied Mechanics, vol. 85, no. 3, 2014, pp. 339–345., doi:10.1007/s00419-014-0957-4.
- Fujimoto, A, et al. “Negative magnetoresistance in Ti-Cleaned single-Layer graphene.” Journal of Physics: Conference Series, vol. 603, 2015, p. 012021., doi:10.1088/1742-6596/603/1/012021.
- Thareja, Vrinda, et al. “Electrically Tunable Coherent Optical Absorption in Graphene with Ion Gel.” Nano Letters, vol. 15, no. 3, 2015, pp. 1570–1576., doi:10.1021/nl503431d.
- Ye, X. H., et al. “Corrosion resistance of graphene directly and locally grown on bulk nickel substrate by laser irradiation.” RSC Advances, vol. 5, no. 45, 2015, pp. 35384–35390., doi:10.1039/c5ra01267h.
- Horvath, Cameron . “Fabrication and Characterization of Edge-Conformed Graphene-Silicon Waveguides.” IEEE Photonics Technology Letters, vol. 27, no. 6, pp. 585–587., doi:10.1109/LPT.2014.2385757.
- Marta, Bogdan, et al. “Efficient etching-Free transfer of high quality, large-Area CVD grown graphene onto polyvinyl alcohol films.” Applied Surface Science, vol. 363, 2016, pp. 613–618., doi:10.1016/j.apsusc.2015.11.265.
- Zheng, Guanpeng, et al. “Improved Transfer Quality of CVD-Grown Graphene by Ultrasonic Processing of Target Substrates: Applications for Ultra-Fast Laser Photonics.” ACS Applied Materials & Interfaces, vol. 5, no. 20, Sept. 2013, pp. 10288–10293., doi:10.1021/am403205v.
- Zeng, Yong, et al. “Investigate the interface structure and growth mechanism of high quality ZnO films grown on multilayer graphene layers.” Applied Surface Science, vol. 301, 2014, pp. 391–395., doi:10.1016/j.apsusc.2014.02.088.
- Ye, Qing, et al. “Polarization-Dependent optical absorption of graphene under total internal reflection.” Applied Physics Letters, vol. 102, no. 2, Jan. 2013, doi:10.1063/1.4776694.
- Watanabe, Hiroshi, et al. “Layer number dependence of carrier lifetime in graphenes observed using time-Resolved mid-Infrared luminescence.” Chemical Physics Letters, vol. 637, 2015, pp. 58–62., doi:10.1016/j.cplett.2015.07.046.
- He, Yingbo, et al. “Strongly enhanced Raman scattering of graphene by a single gold nanorod.” Applied Physics Letters, vol. 107, no. 5, Mar. 2015, p. 053104., doi:10.1063/1.4927759.
- Choi, Duyoung, et al. “Uniformly Nanopatterned Graphene Field-Effect Transistors with Enhanced Properties.” Nanoscale Research Letters, vol. 10, no. 1, Nov. 2015, doi:10.1186/s11671-015-0976-2.
- Miao, Lili, et al. “Broadband ultrafast nonlinear optical response of few-Layers graphene: toward the mid-Infrared regime.” Photonics Research, vol. 3, no. 5, 2015, p. 214., doi:10.1364/prj.3.000214.
- Hu, Jianchen, et al. “Improvement of the electrical contact resistance at rough interfaces using two dimensional materials.” Journal of Applied Physics, vol. 118, no. 21, July 2015, p. 215301., doi:10.1063/1.4936366.
- Jain, Tarun, et al. “Heterogeneous sub-Continuum ionic transport in statistically isolated graphene nanopores.” Nature Nanotechnology, vol. 10, no. 12, May 2015, pp. 1053–1057., doi:10.1038/nnano.2015.222.
- Srisonphan, Siwapon, and Komsan Hongesombut. “Tuning the ballistic electron transport of spatial graphene–metal sandwich electrode on a vacuum-Silicon-Based device.” RSC Advances, vol. 5, no. 3, 2015, pp. 2032–2037., doi:10.1039/c4ra09503k.
- Liu, Xiangjiang, et al. “Compact Shielding of Graphene Monolayer Leads to Extraordinary SERS-Active Substrate with Large-Area Uniformity and Long-Term Stability.” Scientific Reports, vol. 5, no. 1, 2015, doi:10.1038/srep17167.
- Hui, Fei, et al. “Moving graphene devices from lab to market: advanced graphene-Coated nanoprobes.” Nanoscale, vol. 8, no. 16, 2016, pp. 8466–8473., doi:10.1039/c5nr06235g.
- Ye, Xiaohui, et al. “Protecting carbon steel from corrosion by laser in situ grown graphene films.” Carbon, vol. 94, 2015, pp. 326–334., doi:10.1016/j.carbon.2015.06.080.
- Wu, Man, et al. “Wavelength switchable graphene Q-Switched fiber laser with cascaded fiber Bragg gratings.” Optics Communications, vol. 368, 2016, pp. 81–85., doi:10.1016/j.optcom.2016.01.069.
- Mackin, Charles, and Tomás Palacios. “Large-Scale sensor systems based on graphene electrolyte-Gated field-Effect transistors.” The Analyst, vol. 141, no. 9, 2016, pp. 2704–2711., doi:10.1039/c5an02328a.
- Politou, Maria, et al. “Multi-Layer graphene interconnect.” 2016 IEEE International Interconnect Technology Conference / Advanced Metallization Conference (IITC/AMC), July 2016, doi:10.1109/IITC-AMC.2016.7507731.
- Cao, Zhengmin, et al. “Nano-Gap between a gold tip and nanorod for polarization dependent surface enhanced Raman scattering.” Applied Physics Letters, vol. 109, no. 23, May 2016, p. 233103., doi:10.1063/1.4971832.
- Deng, Xiangquan, et al. “Terahertz-Induced photothermoelectric response in graphene-Metal contact structures.” Journal of Physics D: Applied Physics, vol. 49, no. 42, 2016, p. 425101., doi:10.1088/0022-3727/49/42/425101.
- Guo, Chu-Cai, et al. “Experimental Demonstration of Total Absorption over 99% in the Near Infrared for Monolayer-Graphene-Based Subwavelength Structures.” Advanced Optical Materials, vol. 4, no. 12, Jan. 2016, pp. 1955–1960., doi:10.1002/adom.201600481.
- Hu, Jianbo, et al. “Rippling ultrafast dynamics of suspended 2D monolayers, graphene.” Proceedings of the National Academy of Sciences, vol. 113, no. 43, Oct. 2016, doi:10.1073/pnas.1613818113.
- Li, Cheng, et al. “Measurement of the Adhesion Energy of Pressurized Graphene Diaphragm Using Optical Fiber Fabry–Perot Interference.” IEEE Sensors Journal, vol. 16, no. 10, 2016, pp. 3664–3669., doi:10.1109/jsen.2016.2536783.
- Yuan, Jun, et al. “Modulation of far-Infrared light transmission by graphene-Silicon Schottky junction.” Optical Materials Express, vol. 6, no. 12, 2016, p. 3908., doi:10.1364/ome.6.003908.
- Li, Cheng, et al. “Measurement of thermal expansion coefficient of graphene diaphragm using optical fiber Fabry–Perot interference.” Measurement Science and Technology, vol. 27, no. 7, 2016, p. 075102., doi:10.1088/0957-0233/27/7/075102.
- Kafiah, Feras M., et al. “Monolayer graphene transfer onto polypropylene and polyvinylidenedifluoride microfiltration membranes for water desalination.” Desalination, vol. 388, 2016, pp. 29–37., doi:10.1016/j.desal.2016.02.027.
- Wu, Xiangyu, et al. “Doping of graphene for the application in nano-Interconnect.” Microelectronic Engineering, vol. 167, 5 Jan. 2017, pp. 42–46., doi:10.1016/j.mee.2016.10.013.
- Liu, Siyang, et al. “Atomic emission spectroscopy of electrically triggered exploding nanoparticle analytes on graphene/SiO2/Si substrate.” Journal of Vacuum Science & Technology B, Nanotechnology and Microelectronics: Materials, Processing, Measurement, and Phenomena, vol. 34, no. 6, 2016, doi:10.1116/1.4964819.
- Sattler, Klaus D. Carbon nanomaterials sourcebook. Vol. 1, CRC Press, 2016.
- Politou, Maria, et al. “Evaluation of multilayer graphene for advanced interconnects.” Microelectronic Engineering, vol. 167, 5 Jan. 2017, pp. 1–5., doi:10.1016/j.mee.2016.09.011.
- Hwang, Michael T., et al. “Highly specific SNP detection using 2D graphene electronics and DNA strand displacement.” Proceedings of the National Academy of Sciences, vol. 113, no. 26, 2016, pp. 7088–7093., doi:10.1073/pnas.1603753113.
- Nieto, Andy. “Graphene reinforced metal and ceramic matrix composites: a review.” International Materials Reviews, vol. 62, no. 5, 27 Oct. 2017, pp. 241–302., doi:10.1080/09506608.2016.1219481.
- Khorasaninejad, M., et al. “Highly Enhanced Raman Scattering of Graphene using Plasmonic Nano-Structure.” Scientific Reports, vol. 3, no. 1, 2013, doi:10.1038/srep02936.
- Kunz, Daniel A., et al. “Space-Resolved In-Plane Moduli of Graphene Oxide and Chemically Derived Graphene Applying a Simple Wrinkling Procedure (Adv. Mater. 9/2013).” Advanced Materials, vol. 25, no. 9, Apr. 2013, pp. 1336–1336., doi:10.1002/adma.201370060.
- Peng, Shuhua, et al. “Microwetting of Supported Graphene on Hydrophobic Surfaces Revealed by Polymerized Interfacial Femtodroplets.” Langmuir, vol. 30, no. 33, 2014, pp. 10043–10049., doi:10.1021/la5022774.
- Luo, Wen, et al. “Single-Layer Graphene as an Effective Mediator of the Metal–Support Interaction.” The Journal of Physical Chemistry Letters, vol. 5, no. 11, 2014, pp. 1837–1844., doi:10.1021/jz500425j.
- Suemoto, Tohru, et al. “Thickness dependent hot-Phonon effects observed by femtosecond mid-Infrared luminescence in graphene.” 19th International Conference on Ultrafast Phenomena, 2014, doi:10.1364/up.2014.07.mon.p1.32.
- Zhou, Haosen, et al. “Influence of CO2 On the Stability of Discharge Performance for Li-Air Battery with Hybrid Electrolyte Based On the Graphene Sheet.” Conference: 224th ECS Meeting. Oct. 2013, doi:10.1039/c3ra47258b.
- Thareja, Vrinda. “Electrically Tunable Optical Absorption in a Graphene-Based Salisbury Screen.” CORNELL UNIVERSITY LIBRARY, 7 Sept. 2014.
- Horvath, Cameron S. “Light Propagation and Photothermal Nonlinearity in Graphene-Si Waveguides .” University of Alberta Libraries , Nov. 2013, doi:10.7939/R3ST1N.
- Li, Cheng, et al. “Interference characteristics in a Fabry–Perot cavity with graphene membrane for optical fiber pressure sensors.” Microsystem Technologies, vol. 21, no. 11, 2014, pp. 2297–2306., doi:10.1007/s00542-014-2333-2.
- Kou, Jun-Long, et al. “Platform for enhanced light–graphene interaction length and miniaturizing fiber stereo devices.” Optica, vol. 1, no. 5, 2014, p. 307., doi:10.1364/optica.1.000307.
- Karnik, Rohit. “Ionic and Molecular Transport Through Graphene Membranes.” Transport and Reactivity of Solutions in Confined Hydrosystems NATO Science for Peace and Security Series C: Environmental Security, Dec. 2013, pp. 95–102., doi:10.1007/978-94-007-7534-3_8.
- Warren, A. J., et al. “In vacuo X-Ray data collection from graphene-Wrapped protein crystals.” Acta Crystallographica Section D STRUCTURAL BIOLOGY, vol. D71, 2015, pp. 2079–2088., doi:10.1107/S1399004715014194.
- Wen, Chenyu, et al. “Assessing kinetics of surface adsorption–desorption of gas molecules via electrical measurements.” Sensors and Actuators B: Chemical, vol. 223, 2016, pp. 791–798., doi:10.1016/j.snb.2015.10.019.
- Li, Cheng, et al. “Analyzing the applicability of miniature ultra-High sensitivity Fabry–Perot acoustic sensor using a nanothick graphene diaphragm.” Measurement Science and Technology, vol. 26, no. 8, 10 July 2015.
- Li, Cheng, et al. “Analyzing the temperature sensitivity of Fabry-Perot sensor using multilayer graphene diaphragm.” OSA Publishing, vol. 23, no. 21, 2015, pp. 27494–27502., doi:10.1364/OE.23.027494.
- Lau, K. Y., et al. “High Signal-To-Noise Ratio Q-Switching Erbium Doped Fiber Laser Pulse Emission Utilizing Single Layer Trivial Transfer Graphene Film Saturable Absorber.” Jurnal Teknologi, vol. 78, no. 3, 2016, doi:10.11113/jt.v78.7478.
- Zheng, Shijun, et al. “Acoustic charge transport induced by the surface acoustic wave in chemical doped graphene.” Applied Physics Letters, vol. 109, no. 18, 2016, p. 183110., doi:10.1063/1.4967192.
- Deng, Xiangquan, et al. “Terahertz-Induced photothermoelectric response in graphene-Metal contact structures.” Journal of Physics D: Applied Physics, vol. 49, no. 42, 2016, p. 425101., doi:10.1088/0022-3727/49/42/425101.
- Luo, Wen, and Spyridon Zafeiratos. “Inside Back Cover: Graphene-Coated ZnO and SiO2 as Supports for CoO Nanoparticles with Enhanced Reducibility.” ChemPhysChem, vol. 17, no. 19, Apr. 2016, pp. 3146–3146., doi:10.1002/cphc.201601023.
- Lau, K.Y., et al. “Passively mode-Locked soliton femtosecond pulses employing graphene saturable absorber.” Optics & Laser Technology, vol. 94, 2017, pp. 221–227., doi:10.1016/j.optlastec.2017.03.035.
- Fitri, Meika Aidil, et al. “Fabrication of TiO 2 -Graphene photocatalyst by direct chemical vapor deposition and its anti-Fouling property.” Materials Chemistry and Physics, vol. 198, 2017, pp. 42–48., doi:10.1016/j.matchemphys.2017.05.053.
- Li, Cheng, et al. “Nondestructive andin situdetermination of graphene layers using optical fiber Fabry–Perot interference.” Measurement Science and Technology, vol. 28, no. 2, Dec. 2017, p. 025206., doi:10.1088/1361-6501/aa54f8.
- Gao, Xiangyang, et al. “Measuring Graphene Adhesion on Silicon Substrate by Single and Dual Nanoparticle-Loaded Blister.” Advanced Materials Interfaces, vol. 4, no. 9, 2017, p. 1601023., doi:10.1002/admi.201601023.
- Tang, Xiaoduan, et al. “Five Orders of Magnitude Reduction in Energy Coupling across Corrugated Graphene/Substrate Interfaces.” ACS Applied Materials & Interfaces, vol. 6, no. 4, July 2014, pp. 2809–2818., doi:10.1021/am405388a.
- Morrow, W. K., et al. “(Invited) The Use of Graphene as a Solid State Diffusion Barrier.” ECS Transactions, vol. 61, no. 4, 2014, pp. 371–379., doi:10.1149/06104.0371ecst.
- Zheng, Haisheng, et al. “Ultrafine Pt nanoparticle induced doping/Strain of single layer graphene: experimental corroboration between conduction and Raman characteristics.” Journal of Materials Science: Materials in Electronics, vol. 26, no. 7, 2015, pp. 4746–4753., doi:10.1007/s10854-015-3043-y.
- Blinco , James P, et al. “Spin-Coated Carbon." Chemical Science, no. 9, 2013, pp. 3411–3415., doi:10.1039/C3SC51396C.
- Zheng, H., et al. “Effect of Sub 1-Nm Pt Nanoparticle on the Conduction Properties of Graphene Based Field Effect Transistor.” ECS Transactions, vol. 61, no. 39, Jan. 2014, pp. 1–11., doi:10.1149/06139.0001ecst.
- Mckitterick, Christopher B., et al. “Electron-Phonon cooling in large monolayer graphene devices.” Physical Review B, vol. 93, no. 7, Apr. 2016, doi:10.1103/physrevb.93.075410.
- Chan, Chun Yu. “Graphene based electrical biosensors for the detection of biomolecules.” The Hong Kong Polytechnic University, 2016.
- Pápa, Z., et al. “Spectroscopic ellipsometric investigation of graphene and thin carbon films from the point of view of depolarization effects.” Applied Surface Science, vol. 421, 2017, pp. 714–721., doi:10.1016/j.apsusc.2016.11.231.
- Hussain, Mushtaque, et al. “The improved piezoelectric properties of ZnO nanorods with oxygen plasma treatment on the single layer graphene coated polymer substrate.” Physica status solidi (a), vol. 211, no. 2, Oct. 2014, pp. 455–459., doi:10.1002/pssa.201300330.
- Kafiah, Feras, et al. “Synthesis of Graphene Based Membranes: Effect of Substrate Surface Properties on Monolayer Graphene Transfer.” Materials, vol. 10, no. 1, 2017, p. 86., doi:10.3390/ma10010086.
- Liang, Ji, et al. “Modulation of acousto-Electric current using a hybrid on-Chip AlN SAW/GFET device.” Applied Physics Letters, vol. 110, no. 24, Dec. 2017, p. 243504., doi:10.1063/1.4986481.
- Zhang, Yingjie & Kim, Youngseok & J. Gilbert, Matthew & Mason, Nadya. (2017). Electron transport in strain superlattices of graphene.
- Yan, Xiao-Qing, et al. “Polarization dependence of graphene transient optical response: interplay between incident direction and anisotropic distribution of nonequilibrium carriers.” Journal of the Optical Society of America B, vol. 34, no. 1, 2016, p. 218., doi:10.1364/josab.34.000218.
- Huang, Kun, et al. “Graphene coupled with Pt cubic nanoparticles for high performance, air-Stable graphene-Silicon solar cells.” Nano Energy, vol. 32, 2017, pp. 225–231., doi:10.1016/j.nanoen.2016.12.042.
- Lu, Bingyu, et al. “Roughened cylindrical gold layer with curve graphene coating for enhanced sensitivity of fiber SPR sensor.” 2017 19th International Conference on Solid-State Sensors, Actuators and Microsystems (TRANSDUCERS), 2017, doi:10.1109/transducers.2017.7994461.
- Kafiah, Feras, et al. “Synthesis of Graphene Based Membranes: Effect of Substrate Surface Properties on Monolayer Graphene Transfer.” Materials, vol. 10, no. 1, 2017, p. 86., doi:10.3390/ma10010086.
- Hsu, Wei-Hao, et al. “Low-Energy electron point projection microscopy/Diffraction study of suspended graphene.” Applied Surface Science, vol. 423, 30 Nov. 2017, pp. 266–274., doi:10.1016/j.apsusc.2017.06.148.
- Peng, Shuhua, et al. “Microwetting of Supported Graphene on Hydrophobic Surfaces Revealed by Polymerized Interfacial Femtodroplets.” Langmuir, vol. 30, no. 33, 2014, pp. 10043–10049., doi:10.1021/la5022774.
- Horvath, Cameron, et al. “Edge-Conformed silicon-Graphene waveguides: Fabrication and measurements.” 11th International Conference on Group IV Photonics (GFP), 2014, doi:10.1109/group4.2014.6961953.
- Watanabe, Hiroshi, et al. “Femtosecond midi-Infrared luminescence with hot-Phonon effects in graphenes and graphite.”
- Hassan, Saad. Transparent conductive article.
- Liang, Ji, et al. “Manipulation of carriers in graphene using an on-Chip acoustic wave device.” 2017 IEEE International Ultrasonics Symposium (IUS), 2017, doi:10.1109/ultsym.2017.8092898.
- Luo, Wen, et al. “Interaction of bimetallic PtCo layers with bare and graphene-Covered ZnO(0001) supports.” Surface Science, vol. 669, 2018, pp. 64–70., doi:10.1016/j.susc.2017.11.001.
- Jiang, Wen-Shuai, et al. “Preparation of high-Quality graphene using triggered microwave reduction under an air atmosphere.” Journal of Materials Chemistry C, 2018, doi:10.1039/c7tc03957c.
- Dong, Nannan, et al. “Pressure and Temperature Sensor Based on Graphene Diaphragm and Fiber Bragg Gratings.” IEEE Photonics Technology Letters, 2017, pp. 1–1., doi:10.1109/lpt.2017.2786292.
- Huczko, Andrzej, et al. “Efficient one-Pot combustion synthesis of few-Layered graphene.” Physica status solidi (b), vol. 252, no. 11, 2015, pp. 2412–2417., doi:10.1002/pssb.201552233.
- Lu, Bingyu, et al. “Roughened cylindrical gold layer with curve graphene coating for enhanced sensitivity of fiber SPR sensor.” 2017 19th International Conference on Solid-State Sensors, Actuators and Microsystems (TRANSDUCERS), 2017, doi:10.1109/transducers.2017.7994461.
- Fitri, Meika Aidil, et al. “Fabrication of TiO 2 -Graphene photocatalyst by direct chemical vapor deposition and its anti-Fouling property.” Materials Chemistry and Physics, vol. 198, 2017, pp. 42–48., doi:10.1016/j.matchemphys.2017.05.053.
- Bezryadin, A, et al. “Large energy storage efficiency of the dielectric layer of graphene nanocapacitors.” Nanotechnology, vol. 28, no. 49, 2017, p. 495401., doi:10.1088/1361-6528/aa935c.
- Shi, Cheng, et al. “Metamaterial-Based graphene thermal emitter.” Nano Research, June 2017, doi:10.1007/s12274-017-1922-7.
- Mccaffrey, Debra L., et al. “Mechanism of ion adsorption to aqueous interfaces: Graphene/Water vs. air/Water.” Proceedings of the National Academy of Sciences, vol. 114, no. 51, 2017, pp. 13369–13373., doi:10.1073/pnas.1702760114.
- Zhang, Qin, et al. “Negative differential resistance and hysteresis in graphene-Based organic light-Emitting devices.” Journal of Materials Chemistry C, The Royal Society of Chemistry, 1 Jan. 2018, pubs.rsc.org/en/Content/ArticleLanding/2017/TC/C7TC05148D#!
- Miskin, Marc Z., et al. “Graphene-Based bimorphs for micron-Sized, autonomous origami machines.” Proceedings of the National Academy of Sciences, vol. 115, no. 3, Feb. 2018, pp. 466–470., doi:10.1073/pnas.1712889115.
- Bukola, Saheed, et al. “Selective Proton/Deuteron Transport through Nafion|Graphene|Nafion Sandwich Structures at High Current Density.” Journal of the American Chemical Society, vol. 140, no. 5, 2018, pp. 1743–1752., doi:10.1021/jacs.7b10853.
- Brown, Morgan A., et al. “Graphene Biotransistor Interfaced with a Nitrifying Biofilm.” Environmental Science & Technology Letters, vol. 2, no. 4, Mar. 2015, pp. 118–122., doi:10.1021/acs.estlett.5b00025.
- Woo, Sung Oh, and Winfried Teizer. “The effect of electron induced hydrogenation of graphene on its electrical transport properties.” Applied Physics Letters, vol. 103, no. 4, 2013, p. 041603., doi:10.1063/1.4816475.
- Li, Cheng, et al. “Manipulation of Nonlinear Optical Properties of Graphene Bonded Fiber Devices by Thermally Engineering Fermi-Dirac Distribution.” Advanced Optical Materials, vol. 5, no. 21, 2017, doi:10.1002/adom.201770103.
- Chirayath, V. A, et al. “Positron induced electron emission from graphene.” IOP Science, Journal of Physics: Conference Series.
- Lock, Evgeniya H., et al. Stable IR Transparent Conductive Graphene Hybrid Materials and Methods of Making.
- Beechem, Thomas E., et al. “Self-Heating and Failure in Scalable Graphene Devices.” Scientific Reports, vol. 6, no. 1, Sept. 2016, doi:10.1038/srep26457.
- Ma, Yufeng, and Anni Siitonen. Chemical sensor using molecularly-Imprinted single layer graphene.
- Morrow, Wayne K., et al. “Role of graphene interlayers in mitigating degradation of Ni/Au ohmic contact morphology on p-Type GaN.” Vacuum, vol. 128, 2016, pp. 34–38., doi:10.1016/j.vacuum.2016.03.004.
- Nagamanasa, Kandula Hima, et al. “Liquid-Cell Electron Microscopy of Adsorbed Polymers.” Advanced Materials, vol. 29, no. 41, 2017, p. 1703555., doi:10.1002/adma.201703555.
- Hui, Fei. “Variability of graphene devices fabricated using graphene inks: Atomic force microscope tips.” Surface and Coatings Technology, vol. 320, 25 June 2017, doi:10.1016/j.surfcoat.2016.12.020.
- Chirayath, V A, et al. “Investigation of graphene using low energy positron annihilation induced Doppler broadening spectroscopy.” Journal of Physics: Conference Series, vol. 791, 2017, p. 012032., doi:10.1088/1742-6596/791/1/012032.
- Niu, Tianxiao, et al. “Indentation behavior of the stiffest membrane mounted on a very compliant substrate: Graphene on PDMS.” International Journal of Solids and Structures, vol. 132-133, 2018, pp. 1–8., doi:10.1016/j.ijsolstr.2017.05.038.
- Nashed, Ramy, et al. “Ultra-High Mobility in Dielectrically Pinned CVD Graphene.” IEEE Journal of the Electron Devices Society, vol. 4, no. 6, 2016, pp. 466–472., doi:10.1109/jeds.2016.2595498.
- Niu, Tianxiao, et al. “Fracture behavior of graphene mounted on stretchable substrate.” Carbon, vol. 109, 2016, pp. 852–859., doi:10.1016/j.carbon.2016.08.087.
- Boutilier, Michael S H, et al. “Knudsen effusion through polymer-Coated three-Layer porous graphene membranes.” Nanotechnology, vol. 28, no. 18, Oct. 2017, p. 184003., doi:10.1088/1361-6528/aa680f.
- Du, Feng, et al. “Surface stress of graphene layers supported on soft substrate.” Scientific Reports, vol. 6, no. 1, Nov. 2016, doi:10.1038/srep25653.
- Yokaribas, Volkan, et al. “Strain Gauges Based on CVD Graphene Layers and Exfoliated Graphene Nanoplatelets with Enhanced Reproducibility and Scalability for Large Quantities.” Sensors, vol. 17, no. 12, 2017, p. 2937., doi:10.3390/s17122937.
- Han, Ruirui. "Flexible Thermoelectric Generators and 2-D Graphene pH Sensors for Wireless Sensing in Hot Spring Ecosystem." PhD diss., Arizona State University, 2018.
|
-
FAQ
1. What is the best method to dissolve/etch an AAO Template?
There are two methods that we recommend. Please make the best adjustments based on your own experiment.
Slow Removal: Depending on the microstructure evolution, a slow removal of AAO layer may be preferred. This can be achieved by using 5 wt% phosphate acid at 30º Celsius. Usually, this process is suitable for Single-Pass AAO.
Rapid removal: Use heated 3M NaOH or HCl solution, depending on your specific needs.
2. What's the thickness of the Al substrate?
The Al substrate is 150microns thick.
3. What is the best method for cutting an AAO Template?
The method we recommend involves using a metal ruler, a sharp knife or blade, a clean cloth (specialized cleanroom wipes suggested), and a hard flat surface (glass or steel).
Cutting Method:
1. Cover the hard surface with a cleanroom wipe or clean cloth.
2. Place the AAO sample on the wipe with the Al substrate (back side) facing up.
3. Align the metal ruler parallel to the cut you want to make.
4. Press down firmly on the ruler and slice the AAO sample several times along the edge of the ruler.
5. If the AAO sample is not cut through completely, slowly and carefully bend the template until it comes apart.
6. Finally, the AAO sample can be cleaned by ultrasonic cleaning <30 seconds using acetone, ethanol, isopropyl alcohol or deionized water.
Research Citations of ACS Material Products
- Jaworski, Nazariy, and Nazariy Andrushchak. “A method of nanoporous anodic aluminum oxide structure modeling based on Bezier curves generation.” 2017 14th International Conference The Experience of Designing and Application of CAD Systems in Microelectronics (CADSM), 2017, doi:10.1109/cadsm.2017.7916085.
- Fleming, Evan, Feng Du, Eric Ou, Liming Dai, and Li Shi. "Thermal conductivity of carbon nanotubes grown by catalyst-free chemical vapor deposition in nanopores." Carbon 145 (2019): 195-200.
- Liu, Cong. "Nanoporous materials chemistry: From gas separation to high pressure chemistry." (2017).
- Jaworski, Nazariy, Marek Iwaniec, and Mykhaylo Lobur. "Implementation Features of Fibrous Composite Microlevel Structure Model Construction Based on Bezier Curves Generation and OpenCL Technology Usage." Machine Dynamics Research 41 (2017).
- Fleming, Evan. "Multi-scale investigation of composite materials for enhanced phase change thermal storage systems." PhD diss., 2018.
|
-
Research Citations of ACS Material Products
- Meduri, Kavita. "Carbon-Supported Transition Metal Nanoparticles for Catalytic and Electromagnetic Applications." (2018).
- Meduri, Kavita, Candice Stauffer, Wen Qian, Otto Zietz, Andrew Barnum, Graham O’Brien Johnson, Dimin Fan et al. "Palladium and gold nanoparticles on carbon supports as highly efficient catalysts for effective removal of trichloroethylene." Journal of Materials Research 33, no. 16 (2018): 2404-2413.
|
-
Research Citations of ACS Material Products
- Ghasemi-Kahrizsangi, Salman, et al. “The influence of Al 2 O 3 nanoparticles addition on the microstructure and properties of bauxite self–flowing low-Cement castables.” Ceramics International, vol. 43, no. 12, 2017, pp. 8813–8818., doi:10.1016/j.ceramint.2017.04.013.
|
-
Research Citations of ACS Material Products
- Ghasemi-Kahrizsangi, Salman, et al. “The influence of Al 2 O 3 nanoparticles addition on the microstructure and properties of bauxite self–flowing low-Cement castables.” Ceramics International, vol. 43, no. 12, 2017, pp. 8813–8818., doi:10.1016/j.ceramint.2017.04.013.
|
-
FAQ
1. What is the temperature used for drying this product?
This product is dried by low-temperature freezing method and the drying temperature is about 20 degrees Celsius.
2. What is the processing temperature before deformation occurs?
The processing temperature of Graphene Aerogel before deformation is about 120 degrees Celsius.
3. What is the reference to the specific surface area of this product?
Since our product is unique to each piece, the specific surface area will vary. However, the specific surface area of our Graphene Aerogel can be referenced at approximately around ~180 m2/g
4. Can this product be milled into powder without compromising the structure?
Turning the Graphene Aerogel product into powder will indeed compromise the structure. However, we do recomment to check out our Reduced Graphene Oxide (rGO) and Single Layer Graphene to see if these products could work better according to your needs.
5. How fragile is this material? Does it break easily?
Our Graphene Aerogel product has insufficient elasticity, therefore the product will be fragile enough to easily break with large external force.
Disclaimer: ACS Material LLC believes that the information in this Technical Data Sheet is accurate and represents the best and most current information available to us. ACS Material makes no representations or warranties either express or implied, regarding the suitability of the material for any purpose or the accuracy of the information contained within this document. Accordingly, ACS Material will not be responsible for damages resulting from use of or reliance upon this information.
|
-
Research Citations of ACS Material Products
- Nieto, Andy, et al. “Graphene reinforced metal and ceramic matrix composites: a review.” International Materials Reviews, vol. 62, no. 5, 2016, pp. 241–302., doi:10.1080/09506608.2016.1219481.
- Chand, Rohit, and Suresh Neethirajan. “Microfluidic platform integrated with graphene-Gold nano-Composite aptasensor for one-Step detection of norovirus.” Biosensors and Bioelectronics, vol. 98, 2017, pp. 47–53., doi:10.1016/j.bios.2017.06.026.
- Halim, Willy, Jin-Hong Lee, Sang-Mok Park, Rui Zhang, Snatika Sarkar, Travis O’Neil, Yi-Chen Chiang, and Yong Lak Joo. "Directly Deposited Binder-Free Sulfur Electrode Enabled by Air-Controlled Electrospray Process." ACS Applied Energy Materials (2018).
|
-
FAQ
1. What is the difference between Graphene Dispersion in NMP (Dia:1-3μm), Graphene Dispersion in NMP, and Graphene Dispersion in NMP (Oxygen Reduced)?
The preparation method of graphene dispersion (diameter: 1-3μm) in NMP: Physical exfoliate method is different from the latter two: Electrochemical Cleavage. The diameter of the latter two is different from the first one.
Research Citations of ACS Material Products
- Nieto, Andy, et al. “Graphene reinforced metal and ceramic matrix composites: a review.” International Materials Reviews, vol. 62, no. 5, 2016, pp. 241–302., doi:10.1080/09506608.2016.1219481.
- Chand, Rohit, and Suresh Neethirajan. “Microfluidic platform integrated with graphene-Gold nano-Composite aptasensor for one-Step detection of norovirus.” Biosensors and Bioelectronics, vol. 98, 2017, pp. 47–53., doi:10.1016/j.bios.2017.06.026.
|
-
Research Citations of ACS Material Products
- Nieto, Andy, et al. “Graphene reinforced metal and ceramic matrix composites: a review.” International Materials Reviews, vol. 62, no. 5, 2016, pp. 241–302., doi:10.1080/09506608.2016.1219481.
- Chand, Rohit, and Suresh Neethirajan. “Microfluidic platform integrated with graphene-Gold nano-Composite aptasensor for one-Step detection of norovirus.” Biosensors and Bioelectronics, vol. 98, 2017, pp. 47–53., doi:10.1016/j.bios.2017.06.026.
|
-
Research Citations of ACS Material Products
- Nieto, Andy, et al. “Graphene reinforced metal and ceramic matrix composites: a review.” International Materials Reviews, vol. 62, no. 5, 2016, pp. 241–302., doi:10.1080/09506608.2016.1219481.
- Chand, Rohit, and Suresh Neethirajan. “Microfluidic platform integrated with graphene-Gold nano-Composite aptasensor for one-Step detection of norovirus.” Biosensors and Bioelectronics, vol. 98, 2017, pp. 47–53., doi:10.1016/j.bios.2017.06.026.
- O'Neill, Aidan, et al. “Polymer nanocomposites: In situ polymerization of polyamide 6 in the presence of graphene oxide.” Polymer Composites, 27 May 2015, onlinelibrary.wiley.com/doi/10.1002/pc.23612/abstract.
- Chand, Rohit, and Suresh Neethirajan. “Microfluidic platform integrated with graphene-Gold nano-Composite aptasensor for one-Step detection of norovirus.” Biosensors and Bioelectronics, vol. 98, 2017, pp. 47–53., doi:10.1016/j.bios.2017.06.026.
|
|
-
Q&A
Q: What are the thermal stability of Graphene Nanoplatelets at ambient pressure?
A: GNPs will not oxide below 600 Celsius‚ and they are very stable.
Research Citations of ACS Material Products
- Xu, Peng, et al. “Load transfer and mechanical properties of chemically reduced graphene reinforcements in polymer composites.” Nanotechnology, vol. 23, no. 50, 2012, p. 505713., doi:10.1088/0957-4484/23/50/505713.
- Zhang, Xueping, et al. “Preparation and characterization of Pt nanoparticles supported on modified graphite nanoplatelet using solution blending method.” International Journal of Hydrogen Energy, vol. 38, no. 21, 2013, pp. 8909–8913., doi:10.1016/j.ijhydene.2013.05.038.
- Xia, Gaoqiang, et al. “Highly uniform platinum nanoparticles supported on graphite nanoplatelets as a catalyst for proton exchange membrane fuel cells.” International Journal of Hydrogen Energy, vol. 39, no. 28, 23 Sept. 2014, doi:10.1016/j.ijhydene.2013.08.033.
- Li, Xiguang, et al. “Forced assembly by multilayer coextrusion to create oriented graphene reinforced polymer nanocomposites.” Polymer, vol. 55, no. 1, 2014, pp. 248–257., doi:10.1016/j.polymer.2013.11.025.
- Wang, Xuebin, et al. “Three-Dimensional strutted graphene grown by substrate-Free sugar blowing for high-Power-Density supercapacitors.” Nature Communications, vol. 4, 2013, doi:10.1038/ncomms3905.
- Luan, Xinning, and Ying Wang. “Thermal Annealing and Graphene Modification of Exfoliated Hydrogen Titanate Nanosheets for Enhanced Lithium-Ion Intercalation Properties.” Journal of Materials Science & Technology, vol. 30, no. 9, 2014, pp. 839–846., doi:10.1016/j.jmst.2014.07.003.
- Yilmazoglu, O., et al. “Photocathodes based on graphene nanoplatelet emitters on semi-Insulating GaAs photoswitch.” 2014 27th International Vacuum Nanoelectronics Conference (IVNC), 2014, doi:10.1109/ivnc.2014.6894740.
- Swiderska-Mocek, Agnieszka, and Ewelina Rudnicka. “Lithium–sulphur battery with activated carbon cloth-Sulphur cathode and ionic liquid as electrolyte.” Journal of Power Sources, vol. 273, 2015, pp. 162–167., doi:10.1016/j.jpowsour.2014.09.020.
- Johnson, Drew W. “Characterizations of Nanofluid Heat Transfer Enhancements.” June 2013, doi:10.21236/ada590127.
- Wehnert, F., et al. “Design of multifunctional adhesives by the use of carbon nanoparticles.” Journal of Adhesion Science and Technology, vol. 29, no. 17, 2015, pp. 1849–1859., doi:10.1080/01694243.2015.1014536.
- Li, Mingqi, et al. “Fabrication of graphene nanoplatelets-Supported SiO x -Disordered carbon composite and its application in lithium-Ion batteries.” Journal of Power Sources, vol. 293, 2015, pp. 976–982., doi:10.1016/j.jpowsour.2015.06.019.
- Zhang, Genlei, et al. “Small-Sized and highly dispersed Pt nanoparticles loading on graphite nanoplatelets as an effective catalyst for methanol oxidation.” Nanoscale, vol. 7, no. 22, 2015, pp. 10170–10177., doi:10.1039/c5nr01882j.
- Filippidou, M.K., et al. “A flexible strain sensor made of graphene nanoplatelets/Polydimethylsiloxane nanocomposite.” Microelectronic Engineering, vol. 142, 2015, pp. 7–11., doi:10.1016/j.mee.2015.06.007.
- Zhang, Genlei, et al. “Facile synthesis of graphene nanoplate-Supported porous Pt–Cu alloys with high electrocatalytic properties for methanol oxidation.” Journal of Materials Chemistry A, vol. 4, no. 9, 2016, pp. 3316–3323., doi:10.1039/c5ta09937d.
- Nieto, Andy, et al. “Graphene reinforced metal and ceramic matrix composites: a review.” International Materials Reviews, vol. 62, no. 5, 2016, pp. 241–302., doi:10.1080/09506608.2016.1219481.
- Rashed, A.E., and A.A. El-Moneim. “Two steps synthesis approach of MnO 2 /Graphene nanoplates/Graphite composite electrode for supercapacitor application.” Materials Today Energy, vol. 3, 2017, pp. 24–31., doi:10.1016/j.mtener.2017.02.004.
- Dai, Shengdong, et al. “Biothiol-Mediated synthesis of Pt nanoparticles on graphene nanoplates and their application in methanol electrooxidation.” Journal of Materials Science, vol. 53, no. 1, Jan. 2017, pp. 423–434., doi:10.1007/s10853-017-1508-5.
- Piszczyk, Łukasz, et al. “Elastic polyurethane foams containing graphene nanoplatelets.” Advances in Polymer Technology, 2017, doi:10.1002/adv.21819.
- Yoo, Eunjoo, and Haoshen Zhou. “Carbon Cathodes in Rechargeable Lithium-Oxygen Batteries Based on Double-Lithium-Salt Electrolytes.” ChemSusChem, vol. 9, no. 11, 2016, pp. 1249–1254., doi:10.1002/cssc.201600177.
- Talati, Chetasi, and Eric Padron. “An Exercise in Extrapolation: Clinical Management of Atypical CML, MDS/MPN-Unclassifiable, and MDS/MPN-RS-T.” Current Hematologic Malignancy Reports, vol. 11, no. 6, 2016, pp. 425–433., doi:10.1007/s11899-016-0350-1.
- Gutić, Sanjin. “Primena materijala na bazi grafena u elektrokatalizi i skladištenju energije.” 12 Sept. 2016.
- Nasser, Ali, et al. “Enhancing stability of Co gradient in nano-Structured WC&Ndash;Co functionally graded composites using graphene additives.” Journal of the Ceramic Society of Japan, vol. 124, no. 12, 2016, pp. 1191–1198., doi:10.2109/jcersj2.16148.
- Liu, Biwu, et al. “Iron oxide nanozyme catalyzed synthesis of fluorescent polydopamine for light-up Zn2 Detection.” Nanoscale, vol. 8, no. 28, 2016, pp. 13620–13626., doi:10.1039/c6nr02584f.
- Brcic, Haris. “Investigation of the Rheological Properties of Asphalt Binder Containing Graphene Nanoplatelets.” NTNU, 2016.
- Gabriel Hunter MESA. Graphene enhanced piezoelectric article of manufacture, system and method of energy generator and storage cell .
- Strankowski, Michał, et al. “Morphology, Mechanical and Thermal Properties of Thermoplastic Polyurethane Containing Reduced Graphene Oxide and Graphene Nanoplatelets.” Materials, vol. 11, no. 1, June 2018, p. 82., doi:10.3390/ma11010082.
- Köckritz, Tilo, et al. “Integration of carbon allotropes into polydimethylsiloxane to control the electrical conductivity for novel fields of application.” International Journal of Adhesion and Adhesives, 2017, doi:10.1016/j.ijadhadh.2017.12.001.
- Kumar, Pravir, et al. “Strength of Mg–3%Al alloy in presence of graphene nano-Platelets as reinforcement.” Materials Science and Technology, 2018, pp. 1–10., doi:10.1080/02670836.2018.1424380.
- Negro, E., et al. “Graphene-Supported Au-Ni Carbon Nitride Electrocatalysts for the ORR in Alkaline Environment.” ECS Transactions, vol. 72, no. 29, 2016, pp. 1–14., doi:10.1149/07229.0001ecst.
- El-Kady, Omayma, Hossam M. Yehia, and F. Nouh. "Preparation and characterization of Cu/(WC-TiC-Co)/graphene nano-composites as a suitable material for heat sink by powder metallurgy method." International Journal of Refractory Metals and Hard Materials 79 (2019): 108-114.
- Ranjan, Rachit, Nirmal Kumar Singh, Anand Prakash Jaiswal, and Vivek Bajpai. "Metal matrix nano composites using graphene nano platelets indented on copper particles in aluminium matrix." Indent 60 (2018): 600rpm.
- Tapasztó, Orsolya, Viktor Puchy, Zsolt E. Horváth, Zsolt Fogarassy, Eszter Bódis, Zoltán Károly, Katalin Balázsi, Jan Dusza, and Levente Tapasztó. "The effect of graphene nanoplatelet thickness on the fracture toughness of Si3N4 composites." Ceramics International (2018).
- Lazarević-Pašti, Tamara, Vladan Anićijević, Miloš Baljozović, Dragana Vasić Anićijević, Sanjin Gutić, Vesna Vasić, Natalia V. Skorodumova, and Igor A. Pašti. "The impact of the structure of graphene-based materials on the removal of organophosphorus pesticides from water." Environmental Science: Nano (2018).
- Butmee, Preeyanut, Gamolwan Tumcharern, Pranorm Saejueng, Dalibor Stankovic, Astrid Ortner, Juthamas Jitcharoen, Kurt Kalcher, and Anchalee Samphao. "A direct and sensitive electrochemical sensing platform based on ionic liquid functionalized graphene nanoplatelets for the detection of bisphenol A." Journal of Electroanalytical Chemistry 833 (2019): 370-379.
- Karimi, Samira, Ismaeil Ghasemi, and Foroud Abbassi-Sourki. "A study on the crystallization kinetics of PLLA in the presence of Graphene Oxide and PEG-grafted-Graphene Oxide: Effects on the nucleation and chain mobility." Composites Part B: Engineering 158 (2019): 302-310.
- Boonkaew, Suchanat, Sudkate Chaiyo, Sakda Jampasa, Sirirat Rengpipat, Weena Siangproh, and Orawon Chailapakul. "An origami paper-based electrochemical immunoassay for the C-reactive protein using a screen-printed carbon electrode modified with graphene and gold nanoparticles." Microchimica Acta 186, no. 3 (2019): 153.
- Konecka, Kinga, Mariola Brycht, Andrzej Leniart, and Sławomira Skrzypek. "Development and first application of the edge plane pyrolytic graphite electrode modified with graphene nanoplatelets for highly sensitive voltammetric determination of oxolinic acid." Journal of Electroanalytical Chemistry 826 (2018): 76-83.
- Yehia, Hossam M., F. Nouh, and Omayma El-Kady. "Effect of graphene nano-sheets content and sintering time on the microstructure, coefficient of thermal expansion, and mechanical properties of (Cu/WC–TiC-Co) nano-composites." Journal of Alloys and Compounds 764 (2018): 36-43.
- Sun, Yanyan, Ilya Sinev, Wen Ju, Arno Bergmann, Sören Dresp, Stefanie Kühl, Camillo Spöri et al. "Efficient electrochemical hydrogen peroxide production from molecular oxygen on nitrogen-doped mesoporous carbon catalysts." ACS Catalysis 8, no. 4 (2018): 2844-2856.
- Piszczyk, Łukasz, Paulina Kosmela, and Michał Strankowski. "Elastic polyurethane foams containing graphene nanoplatelets." Advances in Polymer Technology 37, no. 6 (2018): 1625-1634.
- Alam, Fahad, M. Choosri, Tejendra K. Gupta, K. M. Varadarajan, D. Choi, and S. Kumar. "Electrical, mechanical and thermal properties of graphene nanoplatelets reinforced UHMWPE nanocomposites." Materials Science and Engineering: B 241 (2019): 82-91.
- Ranjan, Rachit, Nirmal Kumar Singh, Anand Prakash Jaiswal, and Vivek Bajpai. "Metal matrix nano composites using graphene nano platelets indented on copper particles in aluminium matrix." Indent 60 (2018): 600rpm.
- Gupta, Tejendra K., M. Choosri, K. M. Varadarajan, and S. Kumar. "Self-sensing and mechanical performance of CNT/GNP/UHMWPE biocompatible nanocomposites." Journal of materials science 53, no. 11 (2018): 7939-7952.
- Anas, Muhammad, Muhammad Ali Nasir, Zeeshan Asfar, Saad Nauman, Mehmet Akalin, and Faiz Ahmad. "Structural health monitoring of GFRP laminates using graphene-based smart strain gauges." Journal of the Brazilian Society of Mechanical Sciences and Engineering 40, no. 8 (2018): 397.
- Negro, Enrico, Antoine Bach Delpeuch, Keti Vezzu, Graeme Nawn, Federico Bertasi, Alberto Ansaldo, Vittorio Pellegrini et al. "Toward Pt-free anion-exchange membrane fuel cells: Fe–Sn carbon nitride–graphene core–shell electrocatalysts for the oxygen reduction reaction." Chemistry of Materials 30, no. 8 (2018): 2651-2659.
- Strankowski, Michał. "Effect of Variation of Hard Segment Content and Graphene-Based Nanofiller Concentration on Morphological, Thermal, and Mechanical Properties of Polyurethane Nanocomposites." International Journal of Polymer Science 2018 (2018).
- Chen, Junjie, and Jiecheng Han. "A combination of graphene and graphene nanoplatelets: An effective way to improve thermal conductivity for polymers." Results in Physics 15 (2019): 102803.
- Phuong, M. T., P. V. Trinh, N. V. Tuyen, N. N. Dinh, P. N. Minh, N. D. Dung, and B. H. Thang. "Effect of Graphene Nanoplatelet Concentration on the Thermal Conductivity of Silicone Thermal Grease." Journal of Nano-and Electronic Physics 11, no. 5 (2019).
- Mai, Phuong Thi, Tuan Anh Bui, Hau Van Tran, Trinh Van Pham, Dinh Nang Nguyen, Minh Ngoc Phan, and Thang Hung Bui. "Application of Graphene Silicone Grease in heat dissipation for the Intel Core i5 Processor." JOIV: International Journal on Informatics Visualization 3, no. 2-2 (2019): 222-226.
- Rao, YuanQiao, Ping Cai, Kevin J. Cann, F. David Hussein, Wesley R. Mariott, and Phuong A. Cao. "Supported catalyst with improved flowability." U.S. Patent Application 16/357,699, filed July 18, 2019.
- Zrinski, Ivana, Kingkan Pungjunun, Sanja Martinez, Janez Zavašnik, Dalibor Stanković, Kurt Kalcher, and Eda Mehmeti. "Evaluation of phenolic antioxidant capacity in beverages based on laccase immobilized on screen-printed carbon electrode modified with graphene nanoplatelets and gold nanoparticles." Microchemical Journal 152 (2020): 104282.
- Yaqoob, Basit, Riffat Asim Pasha, Mokhtar Awang, Muhammad Ali Nasir, Azhar Hussain, and Kamran Nazir. "Comparison of Mixing Strategies and Hybrid Ratio Optimization for Mechanical Properties Enhancement of Al-CeO 2-GNP’s Metal Matrix Composite Fabricated by Friction Stir Processing." Metallography, Microstructure, and Analysis 8, no. 4 (2019): 534-544.
- ARAÚJO, Ivana dos Santos. "Desenvolvimento e caracterização de revestimentos nanocompósitos de Ni-Grafeno com propriedades anticorrosivas." Master's thesis, Universidade Federal de Pernambuco, 2019.
|
-
Q&A
Q: What are the thermal stability of Graphene Nanoplatelets at ambient pressure?
A: GNPs will not oxide below 600 Celsius‚ and they are very stable.
Research Citations of ACS Material Products
- Xu, Peng, et al. “Load transfer and mechanical properties of chemically reduced graphene reinforcements in polymer composites.” Nanotechnology, vol. 23, no. 50, 2012, p. 505713., doi:10.1088/0957-4484/23/50/505713.
- Zhang, Xueping, et al. “Preparation and characterization of Pt nanoparticles supported on modified graphite nanoplatelet using solution blending method.” International Journal of Hydrogen Energy, vol. 38, no. 21, 2013, pp. 8909–8913., doi:10.1016/j.ijhydene.2013.05.038.
- Xia, Gaoqiang, et al. “Highly uniform platinum nanoparticles supported on graphite nanoplatelets as a catalyst for proton exchange membrane fuel cells.” International Journal of Hydrogen Energy, vol. 39, no. 28, 23 Sept. 2014, doi:10.1016/j.ijhydene.2013.08.033.
- Li, Xiguang, et al. “Forced assembly by multilayer coextrusion to create oriented graphene reinforced polymer nanocomposites.” Polymer, vol. 55, no. 1, 2014, pp. 248–257., doi:10.1016/j.polymer.2013.11.025.
- Wang, Xuebin, et al. “Three-Dimensional strutted graphene grown by substrate-Free sugar blowing for high-Power-Density supercapacitors.” Nature Communications, vol. 4, 2013, doi:10.1038/ncomms3905.
- Luan, Xinning, and Ying Wang. “Thermal Annealing and Graphene Modification of Exfoliated Hydrogen Titanate Nanosheets for Enhanced Lithium-Ion Intercalation Properties.” Journal of Materials Science & Technology, vol. 30, no. 9, 2014, pp. 839–846., doi:10.1016/j.jmst.2014.07.003.
- Yilmazoglu, O., et al. “Photocathodes based on graphene nanoplatelet emitters on semi-Insulating GaAs photoswitch.” 2014 27th International Vacuum Nanoelectronics Conference (IVNC), 2014, doi:10.1109/ivnc.2014.6894740.
- Swiderska-Mocek, Agnieszka, and Ewelina Rudnicka. “Lithium–sulphur battery with activated carbon cloth-Sulphur cathode and ionic liquid as electrolyte.” Journal of Power Sources, vol. 273, 2015, pp. 162–167., doi:10.1016/j.jpowsour.2014.09.020.
- Johnson, Drew W. “Characterizations of Nanofluid Heat Transfer Enhancements.” June 2013, doi:10.21236/ada590127.
- Wehnert, F., et al. “Design of multifunctional adhesives by the use of carbon nanoparticles.” Journal of Adhesion Science and Technology, vol. 29, no. 17, 2015, pp. 1849–1859., doi:10.1080/01694243.2015.1014536.
- Li, Mingqi, et al. “Fabrication of graphene nanoplatelets-Supported SiO x -Disordered carbon composite and its application in lithium-Ion batteries.” Journal of Power Sources, vol. 293, 2015, pp. 976–982., doi:10.1016/j.jpowsour.2015.06.019.
- Zhang, Genlei, et al. “Small-Sized and highly dispersed Pt nanoparticles loading on graphite nanoplatelets as an effective catalyst for methanol oxidation.” Nanoscale, vol. 7, no. 22, 2015, pp. 10170–10177., doi:10.1039/c5nr01882j.
- Filippidou, M.K., et al. “A flexible strain sensor made of graphene nanoplatelets/Polydimethylsiloxane nanocomposite.” Microelectronic Engineering, vol. 142, 2015, pp. 7–11., doi:10.1016/j.mee.2015.06.007.
- Zhang, Genlei, et al. “Facile synthesis of graphene nanoplate-Supported porous Pt–Cu alloys with high electrocatalytic properties for methanol oxidation.” Journal of Materials Chemistry A, vol. 4, no. 9, 2016, pp. 3316–3323., doi:10.1039/c5ta09937d.
- Nieto, Andy, et al. “Graphene reinforced metal and ceramic matrix composites: a review.” International Materials Reviews, vol. 62, no. 5, 2016, pp. 241–302., doi:10.1080/09506608.2016.1219481.
- Rashed, A.E., and A.A. El-Moneim. “Two steps synthesis approach of MnO 2 /Graphene nanoplates/Graphite composite electrode for supercapacitor application.” Materials Today Energy, vol. 3, 2017, pp. 24–31., doi:10.1016/j.mtener.2017.02.004.
- Dai, Shengdong, et al. “Biothiol-Mediated synthesis of Pt nanoparticles on graphene nanoplates and their application in methanol electrooxidation.” Journal of Materials Science, vol. 53, no. 1, Jan. 2017, pp. 423–434., doi:10.1007/s10853-017-1508-5.
- Piszczyk, Łukasz, et al. “Elastic polyurethane foams containing graphene nanoplatelets.” Advances in Polymer Technology, 2017, doi:10.1002/adv.21819.
- Yoo, Eunjoo, and Haoshen Zhou. “Carbon Cathodes in Rechargeable Lithium-Oxygen Batteries Based on Double-Lithium-Salt Electrolytes.” ChemSusChem, vol. 9, no. 11, 2016, pp. 1249–1254., doi:10.1002/cssc.201600177.
- Talati, Chetasi, and Eric Padron. “An Exercise in Extrapolation: Clinical Management of Atypical CML, MDS/MPN-Unclassifiable, and MDS/MPN-RS-T.” Current Hematologic Malignancy Reports, vol. 11, no. 6, 2016, pp. 425–433., doi:10.1007/s11899-016-0350-1.
- Gutić, Sanjin. “Primena materijala na bazi grafena u elektrokatalizi i skladištenju energije.” 12 Sept. 2016.
- Nasser, Ali, et al. “Enhancing stability of Co gradient in nano-Structured WC&Ndash;Co functionally graded composites using graphene additives.” Journal of the Ceramic Society of Japan, vol. 124, no. 12, 2016, pp. 1191–1198., doi:10.2109/jcersj2.16148.
- Liu, Biwu, et al. “Iron oxide nanozyme catalyzed synthesis of fluorescent polydopamine for light-up Zn2 Detection.” Nanoscale, vol. 8, no. 28, 2016, pp. 13620–13626., doi:10.1039/c6nr02584f.
- Brcic, Haris. “Investigation of the Rheological Properties of Asphalt Binder Containing Graphene Nanoplatelets.” NTNU, 2016.
- Gabriel Hunter MESA. Graphene enhanced piezoelectric article of manufacture, system and method of energy generator and storage cell .
- Strankowski, Michał, et al. “Morphology, Mechanical and Thermal Properties of Thermoplastic Polyurethane Containing Reduced Graphene Oxide and Graphene Nanoplatelets.” Materials, vol. 11, no. 1, June 2018, p. 82., doi:10.3390/ma11010082.
- Köckritz, Tilo, et al. “Integration of carbon allotropes into polydimethylsiloxane to control the electrical conductivity for novel fields of application.” International Journal of Adhesion and Adhesives, 2017, doi:10.1016/j.ijadhadh.2017.12.001.
- Kumar, Pravir, et al. “Strength of Mg–3%Al alloy in presence of graphene nano-Platelets as reinforcement.” Materials Science and Technology, 2018, pp. 1–10., doi:10.1080/02670836.2018.1424380.
- Negro, E., et al. “Graphene-Supported Au-Ni Carbon Nitride Electrocatalysts for the ORR in Alkaline Environment.” ECS Transactions, vol. 72, no. 29, 2016, pp. 1–14., doi:10.1149/07229.0001ecst.
- El-Kady, Omayma, Hossam M. Yehia, and F. Nouh. "Preparation and characterization of Cu/(WC-TiC-Co)/graphene nano-composites as a suitable material for heat sink by powder metallurgy method." International Journal of Refractory Metals and Hard Materials 79 (2019): 108-114.
- Ranjan, Rachit, Nirmal Kumar Singh, Anand Prakash Jaiswal, and Vivek Bajpai. "Metal matrix nano composites using graphene nano platelets indented on copper particles in aluminium matrix." Indent 60 (2018): 600rpm.
- Tapasztó, Orsolya, Viktor Puchy, Zsolt E. Horváth, Zsolt Fogarassy, Eszter Bódis, Zoltán Károly, Katalin Balázsi, Jan Dusza, and Levente Tapasztó. "The effect of graphene nanoplatelet thickness on the fracture toughness of Si3N4 composites." Ceramics International (2018).
- Lazarević-Pašti, Tamara, Vladan Anićijević, Miloš Baljozović, Dragana Vasić Anićijević, Sanjin Gutić, Vesna Vasić, Natalia V. Skorodumova, and Igor A. Pašti. "The impact of the structure of graphene-based materials on the removal of organophosphorus pesticides from water." Environmental Science: Nano (2018).
- Butmee, Preeyanut, Gamolwan Tumcharern, Pranorm Saejueng, Dalibor Stankovic, Astrid Ortner, Juthamas Jitcharoen, Kurt Kalcher, and Anchalee Samphao. "A direct and sensitive electrochemical sensing platform based on ionic liquid functionalized graphene nanoplatelets for the detection of bisphenol A." Journal of Electroanalytical Chemistry 833 (2019): 370-379.
- Karimi, Samira, Ismaeil Ghasemi, and Foroud Abbassi-Sourki. "A study on the crystallization kinetics of PLLA in the presence of Graphene Oxide and PEG-grafted-Graphene Oxide: Effects on the nucleation and chain mobility." Composites Part B: Engineering 158 (2019): 302-310.
- Boonkaew, Suchanat, Sudkate Chaiyo, Sakda Jampasa, Sirirat Rengpipat, Weena Siangproh, and Orawon Chailapakul. "An origami paper-based electrochemical immunoassay for the C-reactive protein using a screen-printed carbon electrode modified with graphene and gold nanoparticles." Microchimica Acta 186, no. 3 (2019): 153.
- Konecka, Kinga, Mariola Brycht, Andrzej Leniart, and Sławomira Skrzypek. "Development and first application of the edge plane pyrolytic graphite electrode modified with graphene nanoplatelets for highly sensitive voltammetric determination of oxolinic acid." Journal of Electroanalytical Chemistry 826 (2018): 76-83.
- Yehia, Hossam M., F. Nouh, and Omayma El-Kady. "Effect of graphene nano-sheets content and sintering time on the microstructure, coefficient of thermal expansion, and mechanical properties of (Cu/WC–TiC-Co) nano-composites." Journal of Alloys and Compounds 764 (2018): 36-43.
- Sun, Yanyan, Ilya Sinev, Wen Ju, Arno Bergmann, Sören Dresp, Stefanie Kühl, Camillo Spöri et al. "Efficient electrochemical hydrogen peroxide production from molecular oxygen on nitrogen-doped mesoporous carbon catalysts." ACS Catalysis 8, no. 4 (2018): 2844-2856.
- Piszczyk, Łukasz, Paulina Kosmela, and Michał Strankowski. "Elastic polyurethane foams containing graphene nanoplatelets." Advances in Polymer Technology 37, no. 6 (2018): 1625-1634.
- Alam, Fahad, M. Choosri, Tejendra K. Gupta, K. M. Varadarajan, D. Choi, and S. Kumar. "Electrical, mechanical and thermal properties of graphene nanoplatelets reinforced UHMWPE nanocomposites." Materials Science and Engineering: B 241 (2019): 82-91.
- Ranjan, Rachit, Nirmal Kumar Singh, Anand Prakash Jaiswal, and Vivek Bajpai. "Metal matrix nano composites using graphene nano platelets indented on copper particles in aluminium matrix." Indent 60 (2018): 600rpm.
- Gupta, Tejendra K., M. Choosri, K. M. Varadarajan, and S. Kumar. "Self-sensing and mechanical performance of CNT/GNP/UHMWPE biocompatible nanocomposites." Journal of materials science 53, no. 11 (2018): 7939-7952.
- Anas, Muhammad, Muhammad Ali Nasir, Zeeshan Asfar, Saad Nauman, Mehmet Akalin, and Faiz Ahmad. "Structural health monitoring of GFRP laminates using graphene-based smart strain gauges." Journal of the Brazilian Society of Mechanical Sciences and Engineering 40, no. 8 (2018): 397.
- Negro, Enrico, Antoine Bach Delpeuch, Keti Vezzu, Graeme Nawn, Federico Bertasi, Alberto Ansaldo, Vittorio Pellegrini et al. "Toward Pt-free anion-exchange membrane fuel cells: Fe–Sn carbon nitride–graphene core–shell electrocatalysts for the oxygen reduction reaction." Chemistry of Materials 30, no. 8 (2018): 2651-2659.
- Strankowski, Michał. "Effect of Variation of Hard Segment Content and Graphene-Based Nanofiller Concentration on Morphological, Thermal, and Mechanical Properties of Polyurethane Nanocomposites." International Journal of Polymer Science 2018 (2018).
- Chen, Junjie, and Jiecheng Han. "A combination of graphene and graphene nanoplatelets: An effective way to improve thermal conductivity for polymers." Results in Physics 15 (2019): 102803.
- Phuong, M. T., P. V. Trinh, N. V. Tuyen, N. N. Dinh, P. N. Minh, N. D. Dung, and B. H. Thang. "Effect of Graphene Nanoplatelet Concentration on the Thermal Conductivity of Silicone Thermal Grease." Journal of Nano-and Electronic Physics 11, no. 5 (2019).
- Mai, Phuong Thi, Tuan Anh Bui, Hau Van Tran, Trinh Van Pham, Dinh Nang Nguyen, Minh Ngoc Phan, and Thang Hung Bui. "Application of Graphene Silicone Grease in heat dissipation for the Intel Core i5 Processor." JOIV: International Journal on Informatics Visualization 3, no. 2-2 (2019): 222-226.
- Rao, YuanQiao, Ping Cai, Kevin J. Cann, F. David Hussein, Wesley R. Mariott, and Phuong A. Cao. "Supported catalyst with improved flowability." U.S. Patent Application 16/357,699, filed July 18, 2019.
- Zrinski, Ivana, Kingkan Pungjunun, Sanja Martinez, Janez Zavašnik, Dalibor Stanković, Kurt Kalcher, and Eda Mehmeti. "Evaluation of phenolic antioxidant capacity in beverages based on laccase immobilized on screen-printed carbon electrode modified with graphene nanoplatelets and gold nanoparticles." Microchemical Journal 152 (2020): 104282.
- Yaqoob, Basit, Riffat Asim Pasha, Mokhtar Awang, Muhammad Ali Nasir, Azhar Hussain, and Kamran Nazir. "Comparison of Mixing Strategies and Hybrid Ratio Optimization for Mechanical Properties Enhancement of Al-CeO 2-GNP’s Metal Matrix Composite Fabricated by Friction Stir Processing." Metallography, Microstructure, and Analysis 8, no. 4 (2019): 534-544.
- ARAÚJO, Ivana dos Santos. "Desenvolvimento e caracterização de revestimentos nanocompósitos de Ni-Grafeno com propriedades anticorrosivas." Master's thesis, Universidade Federal de Pernambuco, 2019.
|
-
Q&A
1. What is the thermal stability of Graphene Nanoplatelets at ambient pressure?
GNPs will not oxidize below 600 Celsius‚ they are very stable.
2. Does this product contain any Phosphorus or Sulfate?
There’s no Sulfate, but there may be a little bit of phosphorus, which comes from the raw materials. The exact content of phosphorus has not been tested.
3.What is the particle size distribution of Graphene Nanoplatelets(2-10nm)?
D10=13.56μm, D50=48.93μm, D90=122.2μm
4. How is the surface area for this product determined?
It’s calculated by BET equation.
Research Citations of ACS Material Products
- Xu, Peng, et al. “Load transfer and mechanical properties of chemically reduced graphene reinforcements in polymer composites.” Nanotechnology, vol. 23, no. 50, 2012, p. 505713., doi:10.1088/0957-4484/23/50/505713.
- Zhang, Xueping, et al. “Preparation and characterization of Pt nanoparticles supported on modified graphite nanoplatelet using solution blending method.” International Journal of Hydrogen Energy, vol. 38, no. 21, 2013, pp. 8909–8913., doi:10.1016/j.ijhydene.2013.05.038.
- Xia, Gaoqiang, et al. “Highly uniform platinum nanoparticles supported on graphite nanoplatelets as a catalyst for proton exchange membrane fuel cells.” International Journal of Hydrogen Energy, vol. 39, no. 28, 23 Sept. 2014, doi:10.1016/j.ijhydene.2013.08.033.
- Li, Xiguang, et al. “Forced assembly by multilayer coextrusion to create oriented graphene reinforced polymer nanocomposites.” Polymer, vol. 55, no. 1, 2014, pp. 248–257., doi:10.1016/j.polymer.2013.11.025.
- Wang, Xuebin, et al. “Three-Dimensional strutted graphene grown by substrate-Free sugar blowing for high-Power-Density supercapacitors.” Nature Communications, vol. 4, 2013, doi:10.1038/ncomms3905.
- Luan, Xinning, and Ying Wang. “Thermal Annealing and Graphene Modification of Exfoliated Hydrogen Titanate Nanosheets for Enhanced Lithium-Ion Intercalation Properties.” Journal of Materials Science & Technology, vol. 30, no. 9, 2014, pp. 839–846., doi:10.1016/j.jmst.2014.07.003.
- Yilmazoglu, O., et al. “Photocathodes based on graphene nanoplatelet emitters on semi-Insulating GaAs photoswitch.” 2014 27th International Vacuum Nanoelectronics Conference (IVNC), 2014, doi:10.1109/ivnc.2014.6894740.
- Swiderska-Mocek, Agnieszka, and Ewelina Rudnicka. “Lithium–sulphur battery with activated carbon cloth-Sulphur cathode and ionic liquid as electrolyte.” Journal of Power Sources, vol. 273, 2015, pp. 162–167., doi:10.1016/j.jpowsour.2014.09.020.
- Johnson, Drew W. “Characterizations of Nanofluid Heat Transfer Enhancements.” June 2013, doi:10.21236/ada590127.
- Wehnert, F., et al. “Design of multifunctional adhesives by the use of carbon nanoparticles.” Journal of Adhesion Science and Technology, vol. 29, no. 17, 2015, pp. 1849–1859., doi:10.1080/01694243.2015.1014536.
- Li, Mingqi, et al. “Fabrication of graphene nanoplatelets-Supported SiO x -Disordered carbon composite and its application in lithium-Ion batteries.” Journal of Power Sources, vol. 293, 2015, pp. 976–982., doi:10.1016/j.jpowsour.2015.06.019.
- Zhang, Genlei, et al. “Small-Sized and highly dispersed Pt nanoparticles loading on graphite nanoplatelets as an effective catalyst for methanol oxidation.” Nanoscale, vol. 7, no. 22, 2015, pp. 10170–10177., doi:10.1039/c5nr01882j.
- Filippidou, M.K., et al. “A flexible strain sensor made of graphene nanoplatelets/Polydimethylsiloxane nanocomposite.” Microelectronic Engineering, vol. 142, 2015, pp. 7–11., doi:10.1016/j.mee.2015.06.007.
- Zhang, Genlei, et al. “Facile synthesis of graphene nanoplate-Supported porous Pt–Cu alloys with high electrocatalytic properties for methanol oxidation.” Journal of Materials Chemistry A, vol. 4, no. 9, 2016, pp. 3316–3323., doi:10.1039/c5ta09937d.
- Nieto, Andy, et al. “Graphene reinforced metal and ceramic matrix composites: a review.” International Materials Reviews, vol. 62, no. 5, 2016, pp. 241–302., doi:10.1080/09506608.2016.1219481.
- Rashed, A.E., and A.A. El-Moneim. “Two steps synthesis approach of MnO 2 /Graphene nanoplates/Graphite composite electrode for supercapacitor application.” Materials Today Energy, vol. 3, 2017, pp. 24–31., doi:10.1016/j.mtener.2017.02.004.
- Dai, Shengdong, et al. “Biothiol-Mediated synthesis of Pt nanoparticles on graphene nanoplates and their application in methanol electrooxidation.” Journal of Materials Science, vol. 53, no. 1, Jan. 2017, pp. 423–434., doi:10.1007/s10853-017-1508-5.
- Piszczyk, Łukasz, et al. “Elastic polyurethane foams containing graphene nanoplatelets.” Advances in Polymer Technology, 2017, doi:10.1002/adv.21819.
- Yoo, Eunjoo, and Haoshen Zhou. “Carbon Cathodes in Rechargeable Lithium-Oxygen Batteries Based on Double-Lithium-Salt Electrolytes.” ChemSusChem, vol. 9, no. 11, 2016, pp. 1249–1254., doi:10.1002/cssc.201600177.
- Talati, Chetasi, and Eric Padron. “An Exercise in Extrapolation: Clinical Management of Atypical CML, MDS/MPN-Unclassifiable, and MDS/MPN-RS-T.” Current Hematologic Malignancy Reports, vol. 11, no. 6, 2016, pp. 425–433., doi:10.1007/s11899-016-0350-1.
- Gutić, Sanjin. “Primena materijala na bazi grafena u elektrokatalizi i skladištenju energije.” 12 Sept. 2016.
- Nasser, Ali, et al. “Enhancing stability of Co gradient in nano-Structured WC&Ndash;Co functionally graded composites using graphene additives.” Journal of the Ceramic Society of Japan, vol. 124, no. 12, 2016, pp. 1191–1198., doi:10.2109/jcersj2.16148.
- Liu, Biwu, et al. “Iron oxide nanozyme catalyzed synthesis of fluorescent polydopamine for light-up Zn2 Detection.” Nanoscale, vol. 8, no. 28, 2016, pp. 13620–13626., doi:10.1039/c6nr02584f.
- Brcic, Haris. “Investigation of the Rheological Properties of Asphalt Binder Containing Graphene Nanoplatelets.” NTNU, 2016.
- Gabriel Hunter MESA. Graphene enhanced piezoelectric article of manufacture, system and method of energy generator and storage cell .
- Strankowski, Michał, et al. “Morphology, Mechanical and Thermal Properties of Thermoplastic Polyurethane Containing Reduced Graphene Oxide and Graphene Nanoplatelets.” Materials, vol. 11, no. 1, June 2018, p. 82., doi:10.3390/ma11010082.
- Köckritz, Tilo, et al. “Integration of carbon allotropes into polydimethylsiloxane to control the electrical conductivity for novel fields of application.” International Journal of Adhesion and Adhesives, 2017, doi:10.1016/j.ijadhadh.2017.12.001.
- Kumar, Pravir, et al. “Strength of Mg–3%Al alloy in presence of graphene nano-Platelets as reinforcement.” Materials Science and Technology, 2018, pp. 1–10., doi:10.1080/02670836.2018.1424380.
- Negro, E., et al. “Graphene-Supported Au-Ni Carbon Nitride Electrocatalysts for the ORR in Alkaline Environment.” ECS Transactions, vol. 72, no. 29, 2016, pp. 1–14., doi:10.1149/07229.0001ecst.
- El-Kady, Omayma, Hossam M. Yehia, and F. Nouh. "Preparation and characterization of Cu/(WC-TiC-Co)/graphene nano-composites as a suitable material for heat sink by powder metallurgy method." International Journal of Refractory Metals and Hard Materials 79 (2019): 108-114.
- Ranjan, Rachit, Nirmal Kumar Singh, Anand Prakash Jaiswal, and Vivek Bajpai. "Metal matrix nano composites using graphene nano platelets indented on copper particles in aluminium matrix." Indent 60 (2018): 600rpm.
- Tapasztó, Orsolya, Viktor Puchy, Zsolt E. Horváth, Zsolt Fogarassy, Eszter Bódis, Zoltán Károly, Katalin Balázsi, Jan Dusza, and Levente Tapasztó. "The effect of graphene nanoplatelet thickness on the fracture toughness of Si3N4 composites." Ceramics International (2018).
- Lazarević-Pašti, Tamara, Vladan Anićijević, Miloš Baljozović, Dragana Vasić Anićijević, Sanjin Gutić, Vesna Vasić, Natalia V. Skorodumova, and Igor A. Pašti. "The impact of the structure of graphene-based materials on the removal of organophosphorus pesticides from water." Environmental Science: Nano (2018).
- Butmee, Preeyanut, Gamolwan Tumcharern, Pranorm Saejueng, Dalibor Stankovic, Astrid Ortner, Juthamas Jitcharoen, Kurt Kalcher, and Anchalee Samphao. "A direct and sensitive electrochemical sensing platform based on ionic liquid functionalized graphene nanoplatelets for the detection of bisphenol A." Journal of Electroanalytical Chemistry 833 (2019): 370-379.
- Karimi, Samira, Ismaeil Ghasemi, and Foroud Abbassi-Sourki. "A study on the crystallization kinetics of PLLA in the presence of Graphene Oxide and PEG-grafted-Graphene Oxide: Effects on the nucleation and chain mobility." Composites Part B: Engineering 158 (2019): 302-310.
- Boonkaew, Suchanat, Sudkate Chaiyo, Sakda Jampasa, Sirirat Rengpipat, Weena Siangproh, and Orawon Chailapakul. "An origami paper-based electrochemical immunoassay for the C-reactive protein using a screen-printed carbon electrode modified with graphene and gold nanoparticles." Microchimica Acta 186, no. 3 (2019): 153.
- Konecka, Kinga, Mariola Brycht, Andrzej Leniart, and Sławomira Skrzypek. "Development and first application of the edge plane pyrolytic graphite electrode modified with graphene nanoplatelets for highly sensitive voltammetric determination of oxolinic acid." Journal of Electroanalytical Chemistry 826 (2018): 76-83.
- Yehia, Hossam M., F. Nouh, and Omayma El-Kady. "Effect of graphene nano-sheets content and sintering time on the microstructure, coefficient of thermal expansion, and mechanical properties of (Cu/WC–TiC-Co) nano-composites." Journal of Alloys and Compounds 764 (2018): 36-43.
- Sun, Yanyan, Ilya Sinev, Wen Ju, Arno Bergmann, Sören Dresp, Stefanie Kühl, Camillo Spöri et al. "Efficient electrochemical hydrogen peroxide production from molecular oxygen on nitrogen-doped mesoporous carbon catalysts." ACS Catalysis 8, no. 4 (2018): 2844-2856.
- Piszczyk, Łukasz, Paulina Kosmela, and Michał Strankowski. "Elastic polyurethane foams containing graphene nanoplatelets." Advances in Polymer Technology 37, no. 6 (2018): 1625-1634.
- Alam, Fahad, M. Choosri, Tejendra K. Gupta, K. M. Varadarajan, D. Choi, and S. Kumar. "Electrical, mechanical and thermal properties of graphene nanoplatelets reinforced UHMWPE nanocomposites." Materials Science and Engineering: B 241 (2019): 82-91.
- Ranjan, Rachit, Nirmal Kumar Singh, Anand Prakash Jaiswal, and Vivek Bajpai. "Metal matrix nano composites using graphene nano platelets indented on copper particles in aluminium matrix." Indent 60 (2018): 600rpm.
- Gupta, Tejendra K., M. Choosri, K. M. Varadarajan, and S. Kumar. "Self-sensing and mechanical performance of CNT/GNP/UHMWPE biocompatible nanocomposites." Journal of materials science 53, no. 11 (2018): 7939-7952.
- Anas, Muhammad, Muhammad Ali Nasir, Zeeshan Asfar, Saad Nauman, Mehmet Akalin, and Faiz Ahmad. "Structural health monitoring of GFRP laminates using graphene-based smart strain gauges." Journal of the Brazilian Society of Mechanical Sciences and Engineering 40, no. 8 (2018): 397.
- Negro, Enrico, Antoine Bach Delpeuch, Keti Vezzu, Graeme Nawn, Federico Bertasi, Alberto Ansaldo, Vittorio Pellegrini et al. "Toward Pt-free anion-exchange membrane fuel cells: Fe–Sn carbon nitride–graphene core–shell electrocatalysts for the oxygen reduction reaction." Chemistry of Materials 30, no. 8 (2018): 2651-2659.
- Strankowski, Michał. "Effect of Variation of Hard Segment Content and Graphene-Based Nanofiller Concentration on Morphological, Thermal, and Mechanical Properties of Polyurethane Nanocomposites." International Journal of Polymer Science 2018 (2018).
- Chen, Junjie, and Jiecheng Han. "A combination of graphene and graphene nanoplatelets: An effective way to improve thermal conductivity for polymers." Results in Physics 15 (2019): 102803.
- Phuong, M. T., P. V. Trinh, N. V. Tuyen, N. N. Dinh, P. N. Minh, N. D. Dung, and B. H. Thang. "Effect of Graphene Nanoplatelet Concentration on the Thermal Conductivity of Silicone Thermal Grease." Journal of Nano-and Electronic Physics 11, no. 5 (2019).
- Mai, Phuong Thi, Tuan Anh Bui, Hau Van Tran, Trinh Van Pham, Dinh Nang Nguyen, Minh Ngoc Phan, and Thang Hung Bui. "Application of Graphene Silicone Grease in heat dissipation for the Intel Core i5 Processor." JOIV: International Journal on Informatics Visualization 3, no. 2-2 (2019): 222-226.
- Rao, YuanQiao, Ping Cai, Kevin J. Cann, F. David Hussein, Wesley R. Mariott, and Phuong A. Cao. "Supported catalyst with improved flowability." U.S. Patent Application 16/357,699, filed July 18, 2019.
- Zrinski, Ivana, Kingkan Pungjunun, Sanja Martinez, Janez Zavašnik, Dalibor Stanković, Kurt Kalcher, and Eda Mehmeti. "Evaluation of phenolic antioxidant capacity in beverages based on laccase immobilized on screen-printed carbon electrode modified with graphene nanoplatelets and gold nanoparticles." Microchemical Journal 152 (2020): 104282.
- Yaqoob, Basit, Riffat Asim Pasha, Mokhtar Awang, Muhammad Ali Nasir, Azhar Hussain, and Kamran Nazir. "Comparison of Mixing Strategies and Hybrid Ratio Optimization for Mechanical Properties Enhancement of Al-CeO 2-GNP’s Metal Matrix Composite Fabricated by Friction Stir Processing." Metallography, Microstructure, and Analysis 8, no. 4 (2019): 534-544.
- ARAÚJO, Ivana dos Santos. "Desenvolvimento e caracterização de revestimentos nanocompósitos de Ni-Grafeno com propriedades anticorrosivas." Master's thesis, Universidade Federal de Pernambuco, 2019.
|
-
FAQ
1. What is the crystallinity of this product?
The crystallinity of our Graphene on Ultra-fine 2000 Mesh Copper TEM Grids is polycrystalline.
2. Does your Graphene on Ultra-fine 2000 Mesh Copper TEM Grids have lattice stipes?
No, our Graphene on Ultra-fine 2000 Mesh Cooper TEM Grids does not have lattice stripes.
3. What is the stacking method of your Graphene Ultra-fine 2000 Mesh Cooper TEM Grids?
Our Graphene Ultra-fine 200 Mesh Cooper TEM Grids is not stacked as our graphene is naturally grown.
|
-
FAQ
1. Can the Graphene Oxide on Ultra-flat Thermal SiO2 Substrate used for AFM studies?
Yes, our Ultra-flat Graphene and Graphene Oxide products were designed for AFM studies and we have many customers using them for such applications.
|
-
FAQ
1. Can this product be used for AFM studies?
Yes, our Ultra-flat Graphene and Graphene Oxide products were designed for AFM studies and we have many customers using them for such applications.
|
-
Research Citations of ACS Material Products
- Nieto, Andy, et al. “Graphene reinforced metal and ceramic matrix composites: a review.” International Materials Reviews, vol. 62, no. 5, 2016, pp. 241–302., doi:10.1080/09506608.2016.1219481.
- Gutić, Sanjin J., et al. “Improved catalysts for hydrogen evolution reaction in alkaline solutions through the electrochemical formation of nickel-Reduced graphene oxide interface.” Physical Chemistry, vol. 19, no. 20, 2017, pp. 13281–13293., doi:10.1039/c7cp01237c.
- Gutić, S.j., et al. “Simple routes for the improvement of hydrogen evolution activity of Ni-Mo catalysts: From sol-Gel derived powder catalysts to graphene supported co-Electrodeposits.” International Journal of Hydrogen Energy, 2017, doi:10.1016/j.ijhydene.2017.11.131.
- Wang, Mei, et al. “Concurrent aggregation and transport of graphene oxide in saturated porous media: Roles of temperature, cation type, and electrolyte concentration.” Environmental Pollution, vol. 235, 2018, pp. 350–357., doi:10.1016/j.envpol.2017.12.063.
- Palmieri, Alessandro, Neil Spinner, Shuai Zhao, and William E. Mustain. "Explaining the role and mechanism of carbon matrices in enhancing reaction reversibility of metal oxide anodes for high performance Li ion batteries." carbon 130 (2018): 515-524.
- Gutić, Sanjin J., Muharema Šabanović, Dino Metarapi, Igor A. Pašti, Fehim Korać, and Slavko V. Mentus. "Electrochemically Synthesized Ni@ reduced Graphene Oxide Composite Catalysts for Hydrogen Evolution in Alkaline Media–the Effects of Graphene Oxide Support." Int. J. Electrochem. Sci 14 (2019): 8532-8543.
- Berning, T.; Bessarabov, D. GOMEA: A Conceptual Design of a Membrane Electrode Assembly for a Proton Exchange Membrane Electrolyzer. Membranes 2023, 13, 614. https://doi.org/10.3390/membranes13070614
|
-
Research Citations of ACS Material Products
- Nieto, Andy, et al. “Graphene reinforced metal and ceramic matrix composites: a review.” International Materials Reviews, vol. 62, no. 5, 2016, pp. 241–302., doi:10.1080/09506608.2016.1219481.
|
-
FAQ
1. Is the 'mesh number' listed on the table (positive) + mesh or (negative)–mesh?
The 'mesh number' refers to (positive) + mesh.
2. What is the 0.1% impurities and their makeup?
The 0.1% impurities of our Graphite Flake product contains mainly some volatile matter/components and ash residues such as moisture, carbonate, etc.
3. Is this product artificial graphite?
Our Graphite Flake is natural graphite with fluoride.
|
-
FAQ
1. What is the density specs for this product?
The density of our Graphite Fluoride (Carbon Monofluoride) is at approximately about ~0.35058g/cm3
2. Is Graphite Fluoride (Carbon Monofluoride) artificial graphite?
The Graphite Fluoride (Carbon Monofluoride) is artificial graphite, which is obtained by graphitization of carbon materials.
Research Citations of ACS Material Product
- Lewandowski, Andrzej, and Paweł Jakobczyk. “Kinetics of Na|CF x and Li|CF x systems.” Journal of Solid State Electrochemistry, vol. 20, no. 12, 2016, pp. 3367–3373., doi:10.1007/s10008-016-3305-5.
- Nieto, Andy, et al. “Graphene reinforced metal and ceramic matrix composites: a review.” International Materials Reviews, vol. 62, no. 5, 2016, pp. 241–302., doi:10.1080/09506608.2016.1219481.
- Siyal, Muhammad Irfan, and Jong-Oh Kim. "Fluorographite-co-polydimethylsiloxane coated polyvinylidene-fluoride membrane for desalination of highly saline water with humic acid in direct contact membrane distillation." Environmental research 167 (2018): 255-266.
- Vu, Minh Canh, Nhat Anh Thi Thieu, Jung-Hyurk Lim, Won-Kook Choi, Jong Chan Won, Md Akhtarul Islam, and Sung-Ryong Kim. "Ultrathin thermally conductive yet electrically insulating exfoliated graphene fluoride film for high performance heat dissipation." Carbon (2019).
|
-
Research Citations of ACS Material Products
- You, Shujie, et al. “Effect of synthesis method on solvation and exfoliation of graphite oxide.” Carbon, vol. 52, 2013, pp. 171–180., doi:10.1016/j.carbon.2012.09.018.
- You, Shujie, et al. “Enormous Lattice Expansion of Hummers Graphite Oxide in Alcohols at Low Temperatures.” ACS Nano, vol. 7, no. 2, 2013, pp. 1395–1399., doi:10.1021/nn3051105.
- Talyzin, Alexandr V., et al. “The structure of graphene oxide membranes in liquid water, ethanol and water–ethanol mixtures.” Nanoscale, vol. 6, no. 1, 2014, pp. 272–281., doi:10.1039/c3nr04631a.
- Farjami, Elaheh, et al. “Evidence for oxygen reduction reaction activity of a Ni(OH)2/Graphene oxide catalyst.” Journal of Materials Chemistry A, vol. 1, no. 48, 2013, p. 15501., doi:10.1039/c3ta13351f.
- Vorobiev, Alexei, et al. “Graphene oxide hydration and solvation: an in situ neutron reflectivity study.” Nanoscale, vol. 6, no. 20, 2014, pp. 12151–12156., doi:10.1039/c4nr03621b.
- Klechikov, Alexey G., et al. “Hydrogen storage in bulk graphene-Related materials.” Microporous and Mesoporous Materials, vol. 210, 2015, pp. 46–51., doi:10.1016/j.micromeso.2015.02.017.
- Korobov, Mikhail V., et al. “Sorption of polar organic solvents and water by graphite oxide: Thermodynamic approach.” Carbon, vol. 102, 2016, pp. 297–303., doi:10.1016/j.carbon.2016.02.070.
- Alhumade, Hesham, et al. “Corrosion inhibition of copper in sodium chloride solution using polyetherimide/Graphene composites.” The Canadian Journal of Chemical Engineering, vol. 94, no. 5, 2016, pp. 896–904., doi:10.1002/cjce.22439.
- Hong, Xinghua, et al. “Graphite oxide paper as a polarizable electrical conductor in the through-Thickness direction.” Carbon, vol. 109, 2016, pp. 874–882., doi:10.1016/j.carbon.2016.08.083.
- Nieto, Andy, et al. “Graphene reinforced metal and ceramic matrix composites: a review.” International Materials Reviews, vol. 62, no. 5, 2016, pp. 241–302., doi:10.1080/09506608.2016.1219481.
- Yazdi, Alireza Ahmadian, and Jie Xu. “Nitrogen-Doped graphene approach to enhance the performance of a membraneless enzymatic biofuel cell.” Frontiers in Energy, 2018, doi:10.1007/s11708-018-0529-3.
- Rasmi, K. R., et al. “Synthesis and Characterization of Exfoliated Graphene- and Graphene Oxide-Based Composites.” Graphene and Its Fascinating Attributes, 2011, pp. 33–43., doi:10.1142/9789814329361_0002.
- Chakraborty, Souvik, et al. “Interfacial interaction and the fracture toughness (KIC) trends in epoxy nanocomposites filled with functionalized graphene-Based fillers.” Polymer Composites, 2017, doi:10.1002/pc.24675.
- Yuan, Guohao, et al. “Interconnected ruthenium dioxide nanoparticles anchored on graphite oxide: Highly efficient candidate for solvent-Free oxidative synthesis of imines.” Journal of Industrial and Engineering Chemistry, vol. 46, 2017, pp. 279–288., doi:10.1016/j.jiec.2016.10.040.
- Talyzin, Alexandr V., et al. “Brodie vs Hummers graphite oxides for preparation of multi-Layered materials.” Carbon, vol. 115, 2017, pp. 430–440., doi:10.1016/j.carbon.2016.12.097.
- He, Yibo, Songyan Bai, Zhi Chang, Qi Li, Yu Qiao, and Haoshen Zhou. "Porous hybrid aerogels with ultrahigh sulfur loading for lithium–sulfur batteries." Journal of Materials Chemistry A 6, no. 19 (2018): 9032-9040.
- Hossain, Md Faruk, Jae Sang Heo, John Nelson, and Insoo Kim. "Based Flexible Electrode Using Chemically-Modified Graphene and Functionalized Multiwalled Carbon Nanotube Composites for Electrophysiological Signal Sensing." Information 10, no. 10 (2019): 325.
|
-
FAQ
1. What is the role of DMF in the Green Graphene Quantum Dots in Water?
DMF is an organic solvent with a maximum concentration of 10mg/ml in this product, and there will be a small amount of residue when diluted to 1mg/ml. In addition, the raw material is graphene oxide, which has good dispersibility and does not require additional dispersant. DMF is not a dispersant in the solution.
2. Is there doping in the Green Graphene Quantum Dots in Water?
No.
3. What groups are on the surface of Green Graphene Quantum Dots?
There are a small amount of oxygen groups and amino groups on the surface.
Research Citations of ACS Material Products
- Li, Changzheng, and Yanan Yue. “Fluorescence spectroscopy of graphene quantum dots: temperature effect at different excitation wavelengths.” Nanotechnology, vol. 25, no. 43, Sept. 2014, p. 435703., doi:10.1088/0957-4484/25/43/435703.
- Bhatnagar, Deepika, et al. “Graphene quantum dots FRET based sensor for early detection of heart attack in human.” Biosensors and Bioelectronics, vol. 79, 2016, pp. 495–499., doi:10.1016/j.bios.2015.12.083.
- Liu, Yang, et al. “Electro-Optical switching of liquid crystals sandwiched between ion-Beam-Spurted graphene quantum dots-Doped PEDOT:PSS composite layers.” Optics Express, vol. 23, no. 26, 2015, p. 34071., doi:10.1364/oe.23.034071.
- Bhatnagar, Deepika, et al. “Ultrasensitive cardiac troponin I antibody based nanohybrid sensor for rapid detection of human heart attack.” International Journal of Biological Macromolecules, vol. 95, 2017, pp. 505–510., doi:10.1016/j.ijbiomac.2016.11.037.
- Nieto, Andy, et al. “Graphene reinforced metal and ceramic matrix composites: a review.” International Materials Reviews, vol. 62, no. 5, 2016, pp. 241–302., doi:10.1080/09506608.2016.1219481.
- Bok, Chang Han, et al. “Operating mechanisms of highly-Reproducible write-Once-Read-Many-Times memory devices based on graphene quantum dot:Poly(Methyl silsesquioxane) nanocomposites.” Applied Physics Letters, vol. 110, no. 1, Apr. 2017, p. 013301., doi:10.1063/1.4973358.
- Kim, Do Hyeong, et al. “Highly-Reproducible nonvolatile memristive devices based on polyvinylpyrrolidone: Graphene quantum-Dot nanocomposites.” Organic Electronics, vol. 51, 2017, pp. 156–161., doi:10.1016/j.orgel.2017.09.005.
- Gupta, Shagun, et al. “Ultrasensitive transglutaminase based nanosensor for early detection of celiac disease in human.” International Journal of Biological Macromolecules, vol. 105, 2017, pp. 905–911., doi:10.1016/j.ijbiomac.2017.07.126.
- Ooi, Poh Choon, et al. “Reduced graphene oxide preparation and its applications in solution-Processed write-Once-Read-Many-Times graphene-Based memory device.” Carbon, vol. 124, 2017, pp. 547–554., doi:10.1016/j.carbon.2017.09.004.
- Choi, Hwan Young, et al. “Organic electronic synapses with pinched hystereses based on graphene quantum-Dot nanocomposites.” NPG Asia Materials, vol. 9, no. 7, 2017, doi:10.1038/am.2017.133.
- Ooi, Poh Choon, et al. “Fabrication of transparent bistable switching memory device using plasmapolymerized hexamethyldisiloxane layers with embedded graphene quantum dots.” Thin Solid Films, vol. 645, 2018, pp. 45–50., doi:10.1016/j.tsf.2017.10.044.
- Wang, Changhong, et al. “Computing: Memristive Devices with Highly Repeatable Analog States Boosted by Graphene Quantum Dots.” Small, vol. 13, no. 20, 2017, doi:10.1002/smll.201770110.
- Bakar, Elyani Abu, Mohd Ambri Mohamed, Poh Choon Ooi, MF Mohd Razip Wee, Chang Fu Dee, and Burhanuddin Yeop Majlis. "Fabrication of indium-tin-oxide free, all-solution-processed flexible nanogenerator device using nanocomposite of barium titanate and graphene quantum dots in polyvinylidene fluoride polymer matrix." Organic Electronics 61 (2018): 289-295.
- Ooi, Poh Choon, MF Mohd Razip Wee, Chang Fu Dee, Chi Chin Yap, Muhammad Mat Salleh, and Burhanuddin Yeop Majlis. "Fabrication of transparent bistable switching memory device using plasmapolymerized hexamethyldisiloxane layers with embedded graphene quantum dots." Thin Solid Films 645 (2018): 45-50.
- Sung, Sihyun, Chaoxing Wu, Hyun Soo Jung, and Tae Whan Kim. "Highly-stable write-once-read-many-times switching behaviors of 1D–1R memristive devices based on graphene quantum dot nanocomposites." Scientific reports 8, no. 1 (2018): 12081.
|
-
FAQ
1. Do the sizes refer to the wafer or h-BN coverage or both?
Both. The wafers for this material come in 4, 6 or 8 inch diameter rounds and the h-BN covers 100% of each wafer on one side.
|
-
FAQ
How do I disperse single layer Graphene Oxide into a suitable carrier?
For dispersion in water 1) Use distilled or deionized water 2) Add graphene powder 3) Use sonication power of 200W for about 2 hours
For dispersion in ethanol 1) Use pure ethanol 2) Add graphene powder 3) Use sonication power of 200W for about 4 hours
Is Graphene Oxide fully oxidized? What is the C:O ratio?
Graphene Oxide (GO) is produced by the classic modified Hummer’s method‚ which yields an oxidization level of ≥95%. Regarding the C:O ratio‚ there is some variance inherent in the manufacturing process but the figures below give you an approximation of what to expect:
Sample N (wt %) C (wt %) O (wt %) C/O at. ratio Graphene Oxide 0 51.26 40.78 1.67
NOTE: This graphene oxide with high surface area is easier to be oxidized than the normal graphene oxide‚ and it should be kept out of the sun.
Research Citations of ACS Material Products
- Mukherjee, Rahul, et al. “Photothermally Reduced Graphene as High-Power Anodes for Lithium-Ion Batteries.” ACS Nano, vol. 6, no. 9, 2012, pp. 7867–7878., doi:10.1021/nn303145j.
- Chow, Philippe K., et al. “Mechanical Property Enhancement of Layered Reduced Graphene Oxide Papers by Non-Covalent Modification with Terephthalic Acid.” Particle & Particle Systems Characterization, vol. 31, no. 3, 2013, pp. 337–341., doi:10.1002/ppsc.201300206.
- Feng, Xuefei, et al. “Understanding the degradation mechanism of rechargeable lithium/Sulfur cells: a comprehensive study of the sulfur–graphene oxide cathode after discharge–charge cycling.” Phys. Chem., vol. 16, no. 32, 2014, pp. 16931–16940., doi:10.1039/c4cp01341g.
- Cui, Shumao, et al. “Ultrasensitive Chemical Sensing through Facile Tuning Defects and Functional Groups in Reduced Graphene Oxide.” Analytical Chemistry, vol. 86, no. 15, Nov. 2014, pp. 7516–7522., doi:10.1021/ac501274z.
- Mukherjee, Rahul, et al. “Defect-Induced plating of lithium metal within porous graphene networks.” Nature Communications, vol. 5, 2014, doi:10.1038/ncomms4710.
- Lammel, Tobias, et al. “Internalization and cytotoxicity of graphene oxide and carboxyl graphene nanoplatelets in the human hepatocellular carcinoma cell line Hep G2.” Particle and Fibre Toxicology, vol. 10, no. 1, 2013, p. 27., doi:10.1186/1743-8977-10-27.
- Cheng, Jianli, et al. “Self-Assembled V2O5 nanosheets/Reduced graphene oxide hierarchical nanocomposite as a high-Performance cathode material for lithium ion batteries.” Journal of Materials Chemistry A, vol. 1, no. 36, 2013, p. 10814., doi:10.1039/c3ta12066j.
- Li, Shanghao, et al. “Interaction between Graphene Oxide and Pluronic F127 at the Air–Water Interface.” Langmuir, vol. 29, no. 19, Feb. 2013, pp. 5742–5748., doi:10.1021/la401056t.
- Othman, Abdelhameed M., et al. “Enhancing selectivity in spectrofluorimetric determination of tryptophan by using graphene oxide nanosheets.” Analytica Chimica Acta, vol. 787, 2013, pp. 226–232., doi:10.1016/j.aca.2013.05.036.
- Zhang, Xu, et al. “Effects of Polyethylene Glycol on DNA Adsorption and Hybridization on Gold Nanoparticles and Graphene Oxide.” Langmuir, vol. 28, no. 40, 2012, pp. 14330–14337., doi:10.1021/la302799s.
- Li, Shanghao, et al. “Graphene Oxide as a Quencher for Fluorescent Assay of Amino Acids, Peptides, and Proteins.” ACS Applied Materials & Interfaces, vol. 4, no. 12, Mar. 2012, pp. 7069–7075., doi:10.1021/am302704a.
- Ip, Alexander C.-F., et al. “Oxidation Level-Dependent Zwitterionic Liposome Adsorption and Rupture by Graphene-Based Materials and Light-Induced Content Release.” Small, vol. 9, no. 7, 2012, pp. 1030–1035., doi:10.1002/smll.201202710.
- Chang, Jingbo, et al. “Ultrasonic-Assisted self-Assembly of monolayer graphene oxide for rapid detection of Escherichia coli bacteria.” Nanoscale, vol. 5, no. 9, 2013, p. 3620., doi:10.1039/c3nr00141e.
- Liu, Lin, et al. “Deposition and transport of graphene oxide in saturated and unsaturated porous media.” Chemical Engineering Journal, vol. 229, 1 Aug. 2013, pp. 444–449., doi:10.1016/j.cej.2013.06.030.
- Li, Shanghao, et al. “Head Groups of Lipids Govern the Interaction and Orientation between Graphene Oxide and Lipids.” The Journal of Physical Chemistry C, vol. 117, no. 31, 2013, pp. 16150–16158., doi:10.1021/jp405991q.
- Tran, H. V., et al. “Antibodies Directed to RNA/DNA Hybrids: An Electrochemical Immunosensor for MicroRNAs Detection using Graphene-Composite Electrodes.” Analytical Chemistry, vol. 85, no. 17, 2013, pp. 8469–8474., doi:10.1021/ac402154z.
- Liu, Biwu, et al. “Mechanisms of DNA Sensing on Graphene Oxide.” Analytical Chemistry, vol. 85, no. 16, June 2013, pp. 7987–7993., doi:10.1021/ac401845p.
- Luan, Xinning, et al. “Electrophoretic deposition of reduced graphene oxide nanosheets on TiO2 nanotube arrays for dye-Sensitized solar cells.” Electrochimica Acta, vol. 111, 2013, pp. 216–222., doi:10.1016/j.electacta.2013.08.016.
- Zhang, Ming, et al. “Synthesis of a multifunctional graphene–carbon nanotube aerogel and its strong adsorption of lead from aqueous solution.” RSC Advances, vol. 3, no. 43, 2013, p. 21099., doi:10.1039/c3ra44340j.
- Wu, Lei, et al. “Aggregation Kinetics of Graphene Oxides in Aqueous Solutions: Experiments, Mechanisms, and Modeling.” Langmuir, vol. 29, no. 49, Mar. 2013, pp. 15174–15181., doi:10.1021/la404134x.
- Wang, Feng, and Juewen Liu. “Nanodiamond decorated liposomes as highly biocompatible delivery vehicles and a comparison with carbon nanotubes and graphene oxide.” Nanoscale, vol. 5, no. 24, 2013, p. 12375., doi:10.1039/c3nr04143c.
- Zhang, Ming, et al. “Simple approach for large-Scale production of reduced graphene oxide films.” Chemical Engineering Journal, vol. 243, 2014, pp. 340–346., doi:10.1016/j.cej.2014.01.019.
- Frechette, M. F., et al. “Preparation and dielectric responses of solid epoxy composites containing a mixture of epoxy powder ball-Milled with GO.” 2013 Annual Report Conference on Electrical Insulation and Dielectric Phenomena, 2013, doi:10.1109/ceidp.2013.6748335.
- Chaturvedi, P. et al. “A nanoceria–platinum–graphene nanocomposite for electrochemical biosensing.” Biosensors and Bioelectronics, vol. 58, 15 Aug. 2014, doi:10.1016/j.bios.2014.02.021.
- Lammel, Tobias, and José M. Navas. “Graphene nanoplatelets spontaneously translocate into the cytosol and physically interact with cellular organelles in the fish cell line PLHC-1.” Aquatic Toxicology, vol. 150, 2014, pp. 55–65., doi:10.1016/j.aquatox.2014.02.016.
- Souza, Camille De, et al. “Electrocatalytic miRNA Detection Using Cobalt Porphyrin-Modified Reduced Graphene Oxide.” Sensors, vol. 14, no. 6, June 2014, pp. 9984–9994., doi:10.3390/s140609984.
- Lammel, Tobias, et al. “Potentiating effect of graphene nanomaterials on aromatic environmental pollutant-Induced cytochrome P450 1A expression in the topminnow fish hepatoma cell line PLHC-1.” Environmental Toxicology, vol. 30, no. 10, May 2014, pp. 1192–1204., doi:10.1002/tox.21991.
- Tran, H.V., et al. “An electrochemical ELISA-like immunosensor for miRNAs detection based on screen-Printed gold electrodes modified with reduced graphene oxide and carbon nanotubes.” Biosensors and Bioelectronics, vol. 62, 2014, pp. 25–30., doi:10.1016/j.bios.2014.06.014.
- Wang, Feng, and Juewen Liu. “Platinated DNA oligonucleotides: new probes forming ultrastable conjugates with graphene oxide.” Nanoscale, vol. 6, no. 12, 2014, p. 7079., doi:10.1039/c4nr00867g.
- Chen, Hao, et al. “Removal of sulfamethoxazole and ciprofloxacin from aqueous solutions by graphene oxide.” Journal of Hazardous Materials, vol. 282, 2015, pp. 201–207., doi:10.1016/j.jhazmat.2014.03.063.
- Zhang, Ming, et al. “Slow-Release fertilizer encapsulated by graphene oxide films.” Chemical Engineering Journal, vol. 255, 2014, pp. 107–113., doi:10.1016/j.cej.2014.06.023.
- He, Guang, et al. “Stable Cycling of a Scalable Graphene-Encapsulated Nanocomposite for Lithium–Sulfur Batteries.” ACS Applied Materials & Interfaces, vol. 6, no. 14, May 2014, pp. 10917–10923., doi:10.1021/am500632b.
- Li, Shanghao, et al. “Strong and Selective Adsorption of Lysozyme on Graphene Oxide.” ACS Applied Materials & Interfaces, vol. 6, no. 8, Aug. 2014, pp. 5704–5712., doi:10.1021/am500254e.
- Vanegas, D. C., et al. “Xanthine oxidase biosensor for monitoring meat spoilage.” Smart Biomedical and Physiological Sensor Technology XI, 2014, doi:10.1117/12.2050489.
- Nguyen, Le Huy, et al. “Functionalization of reduced graphene oxide by electroactive polymer for biosensing applications.” Advances in Natural Sciences: Nanoscience and Nanotechnology, vol. 5, no. 3, Sept. 2014, p. 035005., doi:10.1088/2043-6262/5/3/035005.
- Li, Shanghao. “Analysis of the Interactions between Graphene Oxide and Biomolecules and Protein Fibrillation Using Surface Chemistry and Spectroscopy.” University of Miami Scholarly Repository.
- Ding, Zhuhong, et al. “Filtration and transport of heavy metals in graphene oxide enabled sand columns.” Chemical Engineering Journal, vol. 257, 2014, pp. 248–252., doi:10.1016/j.cej.2014.07.034.
- Sims, Christopher M., et al. “CO tolerance of Pt and PtSn intermetallic electrocatalysts on synthetically modified reduced graphene oxide supports.” Dalton Transactions, vol. 44, no. 3, 2015, pp. 977–987., doi:10.1039/c4dt02544j.
- Sun, Yuanyuan, et al. “Transport, retention, and size perturbation of graphene oxide in saturated porous media: Effects of input concentration and grain size.” Water Research, vol. 68, 2015, pp. 24–33., doi:10.1016/j.watres.2014.09.025.
- Feng, Xuefei, et al. “Understanding the degradation mechanism of rechargeable lithium/Sulfur cells: a comprehensive study of the sulfur–graphene oxide cathode after discharge–charge cycling.” Phys. Chem., vol. 16, no. 32, 2014, pp. 16931–16940., doi:10.1039/c4cp01341g.
- Luan, Xinning, et al. “Enhanced photocatalytic activity of graphene oxide/Titania nanosheets composites for methylene blue degradation.” Materials Science in Semiconductor Processing, vol. 30, 2015, pp. 592–598., doi:10.1016/j.mssp.2014.10.032.
- Burrs, S. L. “A comparative study of graphene–hydrogel hybrid bionanocomposites for biosensing.” Analyst, no. 5, 24 Dec. 2014, doi:10.1039/C4AN01788A.
- Tangorra, Rocco Roberto, et al. “Photoactive film by covalent immobilization of a bacterial photosynthetic protein on reduced graphene oxide surface.” MRS Proceedings, vol. 1717, 2015, doi:10.1557/opl.2015.18.
- Huang, Taizhong, et al. “A high-Performance catalyst support for methanol oxidation with graphene and vanadium carbonitride.” Nanoscale, vol. 7, no. 4, 2015, pp. 1301–1307., doi:10.1039/c4nr05244g.
- Chen, Hang, and Tobin Filleter. “Effect of structure on the tribology of ultrathin graphene and graphene oxide films.” Nanotechnology, vol. 26, no. 13.
- Huynh, Vien T., et al. “Polymer coating of graphene oxide via reversible addition-Fragmentation chain transfer mediated emulsion polymerization.” Journal of Polymer Science Part A: Polymer Chemistry, vol. 53, no. 12, 2015, pp. 1413–1421., doi:10.1002/pola.27596.
- Cui, Shumao, et al. “Stabilizing MoS2Nanosheets through SnO2Nanocrystal Decoration for High-Performance Gas Sensing in Air.” Small, vol. 11, no. 19, 2015, pp. 2305–2313., doi:10.1002/smll.201402923.
- Gao, Xianfeng, et al. “A Multilayered Silicon-Reduced Graphene Oxide Electrode for High Performance Lithium-Ion Batteries.” ACS Applied Materials & Interfaces, vol. 7, no. 15, 2015, pp. 7855–7862., doi:10.1021/acsami.5b01230.
- Huang, Po-Jung Jimmy, et al. “Inhibiting the VIM-2 Metallo-β-Lactamase by Graphene Oxide and Carbon Nanotubes.” ACS Applied Materials & Interfaces, vol. 7, no. 18, 2015, pp. 9898–9903., doi:10.1021/acsami.5b01954.
- Zhao, Yingcan, and Chad T. Jafvert. “Environmental photochemistry of single layered graphene oxide in water.” Environmental Science: Nano, vol. 2, no. 2, 2015, pp. 136–142., doi:10.1039/c4en00209a.
- Liang, Xiao, et al. “A highly efficient polysulfide mediator for lithium–sulfur batteries.” Nature Communications, vol. 6, June 2015, p. 5682., doi:10.1038/ncomms6682.
- Vanegas, D. C., et al. “Rapid detection of listeria spp. using an internalin A aptasensor based on carbon-Metal nanohybrid structures.” Smart Biomedical and Physiological Sensor Technology XII, 2015, doi:10.1117/12.2177441.
- Vanegas, Diana C., et al. “A self-Referencing biosensor for real-Time monitoring of physiological ATP transport in plant systems.” Biosensors and Bioelectronics, vol. 74, 2015, pp. 37–44., doi:10.1016/j.bios.2015.05.027.
- Huang, Po-Jung Jimmy, et al. “Hg2 detection using a phosphorothioate RNA probe adsorbed on graphene oxide and a comparison with thymine-Rich DNA.” The Analyst, vol. 141, no. 12, 2016, pp. 3788–3793., doi:10.1039/c5an02031j.
- El-Amin, Ayman A., and Abdelhameed M. Othman. “Novel GO-LaSmO2 Nanocomposite as an Effective Electrode Material for Hydrogen Fuel Cells.” Jom, vol. 68, no. 4, Aug. 2015, pp. 1209–1215., doi:10.1007/s11837-015-1741-9.
- Song, Min-Kyu, et al. “Effects of cell construction parameters on the performance of lithium/Sulfur cells.” AIChE Journal, vol. 61, no. 9, July 2015, pp. 2749–2756., doi:10.1002/aic.14947.
- Dong, Shunan, et al. “Graphene oxide as filter media to remove levofloxacin and lead from aqueous solution.” Chemosphere, vol. 150, 2016, pp. 759–764., doi:10.1016/j.chemosphere.2015.11.075.
- Chang, Jingbo, et al. “Real-Time detection of mercury ions in water using a reduced graphene oxide/DNA field-Effect transistor with assistance of a passivation layer.” Sensing and Bio-Sensing Research, vol. 5, 2015, pp. 97–104., doi:10.1016/j.sbsr.2015.07.009.
- Xu, Wangwang, et al. “Hierarchical Graphene-Encapsulated Hollow SnO2@SnS2 Nanostructures with Enhanced Lithium Storage Capability.” ACS Applied Materials & Interfaces, vol. 7, no. 40, 2015, pp. 22533–22541., doi:10.1021/acsami.5b06765.
- Ye, Yifan. “X-Ray Absorption Spectroscopy Characterization of a Li/S Cell.” Nanomaterials, 2016, doi:10.3390/nano6010014.
- Nguyen, Trang H. D., et al. “Toxicity of Graphene Oxide on Intestinal Bacteria and Caco-2 Cells.” Journal of Food Protection, vol. 78, no. 5, 2015, pp. 996–1002., doi:10.4315/0362-028x.jfp-14-463.
- Huang, Po-Jung Jimmy, et al. “Liposome/Graphene Oxide Interaction Studied by Isothermal Titration Calorimetry.” Langmuir, vol. 32, no. 10, Apr. 2016, pp. 2458–2463., doi:10.1021/acs.langmuir.6b00006.
- Liu, Biwu, et al. “Graphene oxide surface blocking agents can increase the DNA biosensor sensitivity.” Biotechnology Journal, vol. 11, no. 6, Nov. 2016, pp. 780–787., doi:10.1002/biot.201500540.
- Liu, Taoze, et al. “Biochar-Supported carbon nanotube and graphene oxide nanocomposites for Pb(Ii) and Cd(Ii) removal.” RSC Advances, vol. 6, no. 29, 2016, pp. 24314–24319., doi:10.1039/c6ra01895e.
- Nguyen, T. Dung, et al. “One-Step Electrosynthesis of Poly(1,5-Diaminonaphthalene)/Graphene Nanocomposite as Platform for Lead Detection in Water.” Electroanalysis, vol. 28, no. 8, Nov. 2016, pp. 1907–1913., doi:10.1002/elan.201501075.
- Tegou, E., et al. “Low-Temperature thermal reduction of graphene oxide films in ambient atmosphere: Infra-Red spectroscopic studies and gas sensing applications.” Microelectronic Engineering, vol. 159, 2016, pp. 146–150., doi:10.1016/j.mee.2016.03.030.
- Xu, Wangwang, et al. “Direct growth of an economic green energy storage material: a monocrystalline jarosite-KFe3(SO4)2(OH)6-Nanoplates@RGO hybrid as a superior lithium-Ion battery cathode.” Journal of Materials Chemistry A, vol. 4, no. 10, 2016, pp. 3735–3742., doi:10.1039/c5ta10622b.
- Pandey, Gaind P. “Mesoporous Hybrids of Reduced Graphene Oxide and Vanadium Pentoxide for Enhanced Performance in Lithium-Ion Batteries and Electrochemical Capacitors.” Applied Materials & Interfaces, 24 Mar. 2016, pp. 9200–9210., doi:10.1021/acsami.6b02372.
- Nieto, Andy, et al. “Graphene reinforced metal and ceramic matrix composites: a review.” International Materials Reviews, vol. 62, no. 5, 2016, pp. 241–302., doi:10.1080/09506608.2016.1219481.
- Wang, Mei, et al. “Concurrent aggregation and transport of graphene oxide in saturated porous media: Roles of temperature, cation type, and electrolyte concentration.” Environmental Pollution, vol. 235, 2018, pp. 350–357., doi:10.1016/j.envpol.2017.12.063.
- Berning, T.; Bessarabov, D. GOMEA: A Conceptual Design of a Membrane Electrode Assembly for a Proton Exchange Membrane Electrolyzer. Membranes 2023, 13, 614. https://doi.org/10.3390/membranes13070614
|
-
Research Citations of ACS Material Products
- Li, Changzheng, and Yanan Yue. “Fluorescence spectroscopy of graphene quantum dots: temperature effect at different excitation wavelengths.” Nanotechnology, vol. 25, no. 43, Sept. 2014, p. 435703., doi:10.1088/0957-4484/25/43/435703.
- Bhatnagar, Deepika, et al. “Graphene quantum dots FRET based sensor for early detection of heart attack in human.” Biosensors and Bioelectronics, vol. 79, 2016, pp. 495–499., doi:10.1016/j.bios.2015.12.083.
- Liu, Yang, et al. “Electro-Optical switching of liquid crystals sandwiched between ion-Beam-Spurted graphene quantum dots-Doped PEDOT:PSS composite layers.” Optics Express, vol. 23, no. 26, 2015, p. 34071., doi:10.1364/oe.23.034071.
- Bhatnagar, Deepika, et al. “Ultrasensitive cardiac troponin I antibody based nanohybrid sensor for rapid detection of human heart attack.” International Journal of Biological Macromolecules, vol. 95, 2017, pp. 505–510., doi:10.1016/j.ijbiomac.2016.11.037.
- Nieto, Andy, et al. “Graphene reinforced metal and ceramic matrix composites: a review.” International Materials Reviews, vol. 62, no. 5, 2016, pp. 241–302., doi:10.1080/09506608.2016.1219481.
- Bok, Chang Han, et al. “Operating mechanisms of highly-Reproducible write-Once-Read-Many-Times memory devices based on graphene quantum dot:Poly(Methyl silsesquioxane) nanocomposites.” Applied Physics Letters, vol. 110, no. 1, Apr. 2017, p. 013301., doi:10.1063/1.4973358.
- Kim, Do Hyeong, et al. “Highly-Reproducible nonvolatile memristive devices based on polyvinylpyrrolidone: Graphene quantum-Dot nanocomposites.” Organic Electronics, vol. 51, 2017, pp. 156–161., doi:10.1016/j.orgel.2017.09.005.
- Gupta, Shagun, et al. “Ultrasensitive transglutaminase based nanosensor for early detection of celiac disease in human.” International Journal of Biological Macromolecules, vol. 105, 2017, pp. 905–911., doi:10.1016/j.ijbiomac.2017.07.126.
- Ooi, Poh Choon, et al. “Reduced graphene oxide preparation and its applications in solution-Processed write-Once-Read-Many-Times graphene-Based memory device.” Carbon, vol. 124, 2017, pp. 547–554., doi:10.1016/j.carbon.2017.09.004.
- Choi, Hwan Young, et al. “Organic electronic synapses with pinched hystereses based on graphene quantum-Dot nanocomposites.” NPG Asia Materials, vol. 9, no. 7, 2017, doi:10.1038/am.2017.133.
- Ooi, Poh Choon, et al. “Fabrication of transparent bistable switching memory device using plasmapolymerized hexamethyldisiloxane layers with embedded graphene quantum dots.” Thin Solid Films, vol. 645, 2018, pp. 45–50., doi:10.1016/j.tsf.2017.10.044.
- Wang, Changhong, et al. “Computing: Memristive Devices with Highly Repeatable Analog States Boosted by Graphene Quantum Dots.” Small, vol. 13, no. 20, 2017, doi:10.1002/smll.201770110.
- Bakar, Elyani Abu, Mohd Ambri Mohamed, Poh Choon Ooi, MF Mohd Razip Wee, Chang Fu Dee, and Burhanuddin Yeop Majlis. "Fabrication of indium-tin-oxide free, all-solution-processed flexible nanogenerator device using nanocomposite of barium titanate and graphene quantum dots in polyvinylidene fluoride polymer matrix." Organic Electronics 61 (2018): 289-295.
- Ooi, Poh Choon, MF Mohd Razip Wee, Chang Fu Dee, Chi Chin Yap, Muhammad Mat Salleh, and Burhanuddin Yeop Majlis. "Fabrication of transparent bistable switching memory device using plasmapolymerized hexamethyldisiloxane layers with embedded graphene quantum dots." Thin Solid Films 645 (2018): 45-50
- Sung, Sihyun, Chaoxing Wu, Hyun Soo Jung, and Tae Whan Kim. "Highly-stable write-once-read-many-times switching behaviors of 1D–1R memristive devices based on graphene quantum dot nanocomposites." Scientific reports 8, no. 1 (2018): 12081.
|
-
Research Citations of ACS Material Products
- Li, Changzheng, and Yanan Yue. “Fluorescence spectroscopy of graphene quantum dots: temperature effect at different excitation wavelengths.” Nanotechnology, vol. 25, no. 43, Sept. 2014, p. 435703., doi:10.1088/0957-4484/25/43/435703.
- Bhatnagar, Deepika, et al. “Graphene quantum dots FRET based sensor for early detection of heart attack in human.” Biosensors and Bioelectronics, vol. 79, 2016, pp. 495–499., doi:10.1016/j.bios.2015.12.083.
- Liu, Yang, et al. “Electro-Optical switching of liquid crystals sandwiched between ion-Beam-Spurted graphene quantum dots-Doped PEDOT:PSS composite layers.” Optics Express, vol. 23, no. 26, 2015, p. 34071., doi:10.1364/oe.23.034071.
- Bhatnagar, Deepika, et al. “Ultrasensitive cardiac troponin I antibody based nanohybrid sensor for rapid detection of human heart attack.” International Journal of Biological Macromolecules, vol. 95, 2017, pp. 505–510., doi:10.1016/j.ijbiomac.2016.11.037.
- Nieto, Andy, et al. “Graphene reinforced metal and ceramic matrix composites: a review.” International Materials Reviews, vol. 62, no. 5, 2016, pp. 241–302., doi:10.1080/09506608.2016.1219481.
- Bok, Chang Han, et al. “Operating mechanisms of highly-Reproducible write-Once-Read-Many-Times memory devices based on graphene quantum dot:Poly(Methyl silsesquioxane) nanocomposites.” Applied Physics Letters, vol. 110, no. 1, Apr. 2017, p. 013301., doi:10.1063/1.4973358.
- Kim, Do Hyeong, et al. “Highly-Reproducible nonvolatile memristive devices based on polyvinylpyrrolidone: Graphene quantum-Dot nanocomposites.” Organic Electronics, vol. 51, 2017, pp. 156–161., doi:10.1016/j.orgel.2017.09.005.
- Gupta, Shagun, et al. “Ultrasensitive transglutaminase based nanosensor for early detection of celiac disease in human.” International Journal of Biological Macromolecules, vol. 105, 2017, pp. 905–911., doi:10.1016/j.ijbiomac.2017.07.126.
- Ooi, Poh Choon, et al. “Reduced graphene oxide preparation and its applications in solution-Processed write-Once-Read-Many-Times graphene-Based memory device.” Carbon, vol. 124, 2017, pp. 547–554., doi:10.1016/j.carbon.2017.09.004.
- Choi, Hwan Young, et al. “Organic electronic synapses with pinched hystereses based on graphene quantum-Dot nanocomposites.” NPG Asia Materials, vol. 9, no. 7, 2017, doi:10.1038/am.2017.133.
- Ooi, Poh Choon, et al. “Fabrication of transparent bistable switching memory device using plasmapolymerized hexamethyldisiloxane layers with embedded graphene quantum dots.” Thin Solid Films, vol. 645, 2018, pp. 45–50., doi:10.1016/j.tsf.2017.10.044.
- Wang, Changhong, et al. “Computing: Memristive Devices with Highly Repeatable Analog States Boosted by Graphene Quantum Dots.” Small, vol. 13, no. 20, 2017, doi:10.1002/smll.201770110.
- Bakar, Elyani Abu, Mohd Ambri Mohamed, Poh Choon Ooi, MF Mohd Razip Wee, Chang Fu Dee, and Burhanuddin Yeop Majlis. "Fabrication of indium-tin-oxide free, all-solution-processed flexible nanogenerator device using nanocomposite of barium titanate and graphene quantum dots in polyvinylidene fluoride polymer matrix." Organic Electronics 61 (2018): 289-295.
- Ooi, Poh Choon, MF Mohd Razip Wee, Chang Fu Dee, Chi Chin Yap, Muhammad Mat Salleh, and Burhanuddin Yeop Majlis. "Fabrication of transparent bistable switching memory device using plasmapolymerized hexamethyldisiloxane layers with embedded graphene quantum dots." Thin Solid Films 645 (2018): 45-50.
- Sung, Sihyun, Chaoxing Wu, Hyun Soo Jung, and Tae Whan Kim. "Highly-stable write-once-read-many-times switching behaviors of 1D–1R memristive devices based on graphene quantum dot nanocomposites." Scientific reports 8, no. 1 (2018): 12081.
|
-
Research Citations of ACS Material Products
- Li, Changzheng, and Yanan Yue. “Fluorescence spectroscopy of graphene quantum dots: temperature effect at different excitation wavelengths.” Nanotechnology, vol. 25, no. 43, Sept. 2014, p. 435703., doi:10.1088/0957-4484/25/43/435703.
- Bhatnagar, Deepika, et al. “Graphene quantum dots FRET based sensor for early detection of heart attack in human.” Biosensors and Bioelectronics, vol. 79, 2016, pp. 495–499., doi:10.1016/j.bios.2015.12.083.
- Liu, Yang, et al. “Electro-Optical switching of liquid crystals sandwiched between ion-Beam-Spurted graphene quantum dots-Doped PEDOT:PSS composite layers.” Optics Express, vol. 23, no. 26, 2015, p. 34071., doi:10.1364/oe.23.034071.
- Bhatnagar, Deepika, et al. “Ultrasensitive cardiac troponin I antibody based nanohybrid sensor for rapid detection of human heart attack.” International Journal of Biological Macromolecules, vol. 95, 2017, pp. 505–510., doi:10.1016/j.ijbiomac.2016.11.037.
- Nieto, Andy, et al. “Graphene reinforced metal and ceramic matrix composites: a review.” International Materials Reviews, vol. 62, no. 5, 2016, pp. 241–302., doi:10.1080/09506608.2016.1219481.
- Bok, Chang Han, et al. “Operating mechanisms of highly-Reproducible write-Once-Read-Many-Times memory devices based on graphene quantum dot:Poly(Methyl silsesquioxane) nanocomposites.” Applied Physics Letters, vol. 110, no. 1, Apr. 2017, p. 013301., doi:10.1063/1.4973358.
- Kim, Do Hyeong, et al. “Highly-Reproducible nonvolatile memristive devices based on polyvinylpyrrolidone: Graphene quantum-Dot nanocomposites.” Organic Electronics, vol. 51, 2017, pp. 156–161., doi:10.1016/j.orgel.2017.09.005.
- Gupta, Shagun, et al. “Ultrasensitive transglutaminase based nanosensor for early detection of celiac disease in human.” International Journal of Biological Macromolecules, vol. 105, 2017, pp. 905–911., doi:10.1016/j.ijbiomac.2017.07.126.
- Ooi, Poh Choon, et al. “Reduced graphene oxide preparation and its applications in solution-Processed write-Once-Read-Many-Times graphene-Based memory device.” Carbon, vol. 124, 2017, pp. 547–554., doi:10.1016/j.carbon.2017.09.004.
- Choi, Hwan Young, et al. “Organic electronic synapses with pinched hystereses based on graphene quantum-Dot nanocomposites.” NPG Asia Materials, vol. 9, no. 7, 2017, doi:10.1038/am.2017.133.
- Ooi, Poh Choon, et al. “Fabrication of transparent bistable switching memory device using plasmapolymerized hexamethyldisiloxane layers with embedded graphene quantum dots.” Thin Solid Films, vol. 645, 2018, pp. 45–50., doi:10.1016/j.tsf.2017.10.044.
- Wang, Changhong, et al. “Computing: Memristive Devices with Highly Repeatable Analog States Boosted by Graphene Quantum Dots.” Small, vol. 13, no. 20, 2017, doi:10.1002/smll.201770110.
- Bakar, Elyani Abu, Mohd Ambri Mohamed, Poh Choon Ooi, MF Mohd Razip Wee, Chang Fu Dee, and Burhanuddin Yeop Majlis. "Fabrication of indium-tin-oxide free, all-solution-processed flexible nanogenerator device using nanocomposite of barium titanate and graphene quantum dots in polyvinylidene fluoride polymer matrix." Organic Electronics 61 (2018): 289-295.
- Ooi, Poh Choon, MF Mohd Razip Wee, Chang Fu Dee, Chi Chin Yap, Muhammad Mat Salleh, and Burhanuddin Yeop Majlis. "Fabrication of transparent bistable switching memory device using plasmapolymerized hexamethyldisiloxane layers with embedded graphene quantum dots." Thin Solid Films 645 (2018): 45-50.
- Sung, Sihyun, Chaoxing Wu, Hyun Soo Jung, and Tae Whan Kim. "Highly-stable write-once-read-many-times switching behaviors of 1D–1R memristive devices based on graphene quantum dot nanocomposites." Scientific reports 8, no. 1 (2018): 12081.
|
-
Research Citations of ACS Material Products
- Berning, T.; Bessarabov, D. GOMEA: A Conceptual Design of a Membrane Electrode Assembly for a Proton Exchange Membrane Electrolyzer. Membranes 2023, 13, 614. https://doi.org/10.3390/membranes13070614
|
-
Research Citations of ACS Material Products
- Martín, C.f., et al. “Wet impregnation of a commercial low cost silica using DETA for a fast post-Combustion CO 2 capture process.” Applied Energy, vol. 183, 2016, pp. 1705–1721., doi:10.1016/j.apenergy.2016.09.081.
- Dumitriu, Georgiana-Diana, et al. “Study of the potential use of mesoporous nanomaterials as fining agent to prevent protein haze in white wines and its impact in major volatile aroma compounds and polyols.” Food Chemistry, vol. 240, 2018, pp. 751–758., doi:10.1016/j.foodchem.2017.07.163.
- Piumetti, Marco, et al. “Investigations into nanostructured ceria–zirconia catalysts for soot combustion.” Applied Catalysis B: Environmental, vol. 180, 2016, pp. 271–282., doi:10.1016/j.apcatb.2015.06.018.
- Dumitriu, Georgiana-Diana, Nieves López de Lerma, Valeriu V. Cotea, and Rafael A. Peinado. "Application of Mesoporous Materials as Fining Agents for Pedro Ximénez Wines." (2018).
|
-
Research Citations of ACS Material Products
- Berning, T.; Bessarabov, D. GOMEA: A Conceptual Design of a Membrane Electrode Assembly for a Proton Exchange Membrane Electrolyzer. Membranes 2023, 13, 614. https://doi.org/10.3390/membranes13070614
|
-
Research Citations of ACS Material Products
- Meduri, Kavita. "Carbon-Supported Transition Metal Nanoparticles for Catalytic and Electromagnetic Applications." (2018).
- Meduri, Kavita, Candice Stauffer, Wen Qian, Otto Zietz, Andrew Barnum, Graham O’Brien Johnson, Dimin Fan et al. "Palladium and gold nanoparticles on carbon supports as highly efficient catalysts for effective removal of trichloroethylene." Journal of Materials Research 33, no. 16 (2018): 2404-2413.
|
-
Research Citations of ACS Material Products
- Berning, T.; Bessarabov, D. GOMEA: A Conceptual Design of a Membrane Electrode Assembly for a Proton Exchange Membrane Electrolyzer. Membranes 2023, 13, 614. https://doi.org/10.3390/membranes13070614
|
-
Research Citations of ACS Material Products
- Siahaan, Evi Amelia, et al. “Controlled release of allyl isothiocyanate from brown algae Laminaria japonica and mesoporous silica MCM-41 for inhibiting food-Borne bacteria.” Food Science and Biotechnology, vol. 22, no. S1, 2013, pp. 19–24., doi:10.1007/s10068-013-0043-7.
- López-Aranguren, Pedro, et al. “A new method using compressed CO2 for the in situ functionalization of mesoporous silica with hyperbranched polymers.” Chemical Communications, vol. 49, no. 100, 2013, p. 11776., doi:10.1039/c3cc47931e.
- López-Aranguren, P., et al. “Regenerable solid CO2 sorbents prepared by supercritical grafting of aminoalkoxysilane into low-Cost mesoporous silica.” The Journal of Supercritical Fluids, vol. 85, 2014, pp. 68–80., doi:10.1016/j.supflu.2013.10.020.
- López-Aranguren, Pedro, et al. “Understanding the Performance of New Amine-Functionalized Mesoporous Silica Materials for CO2 Adsorption.” Industrial & Engineering Chemistry Research, vol. 53, no. 40, 2014, pp. 15611–15619., doi:10.1021/ie502945r.
- Zandavi, Seyed Hadi, and C. A. Ward. “Nucleation and growth of condensate in nanoporous materials.” Physical Chemistry, vol. 17, no. 15, 2015, pp. 9828–9834., doi:10.1039/c5cp00471c.
- Saliba, Sarmenio, et al. “Combined influence of pore size distribution and surface hydrophilicity on the water adsorption characteristics of micro- and mesoporous silica.” Microporous and Mesoporous Materials, vol. 226, 2016, pp. 221–228., doi:10.1016/j.micromeso.2015.12.029.
- Melnichenko, Yuri B. “Structural Characterization of Porous Materials Using SAS.” Small-Angle Scattering from Confined and Interfacial Fluids, 2016, pp. 139–171., doi:10.1007/978-3-319-01104-2_7.
- Bruzzoniti, M. C., et al. “Adsorption of bentazone herbicide onto mesoporous silica: application to environmental water purification.” Environmental Science and Pollution Research, vol. 23, no. 6, 2015, pp. 5399–5409., doi:10.1007/s11356-015-5755-1.
- Lazdovica, K., et al. “Comparative wheat straw catalytic pyrolysis in the presence of zeolites, Pt/C, and Pd/C by using TGA-FTIR method.” Fuel Processing Technology, vol. 138, 2015, pp. 645–653., doi:10.1016/j.fuproc.2015.07.005.
- Dumitriu, Georgiana-Diana, et al. “Study of the potential use of mesoporous nanomaterials as fining agent to prevent protein haze in white wines and its impact in major volatile aroma compounds and polyols.” Food Chemistry, vol. 240, 2018, pp. 751–758., doi:10.1016/j.foodchem.2017.07.163.
- Dolbin, A. V., et al. “Quantum effects in the sorption kinetics of 4He by mesoporous materials.” Low Temperature Physics, vol. 42, no. 2, 2016, pp. 80–84., doi:10.1063/1.4941598.
- Jabbari-Hichri, Amira, et al. “Effect of aluminum sulfate addition on the thermal storage performance of mesoporous SBA-15 and MCM-41 materials.” Solar Energy Materials and Solar Cells, vol. 149, 2016, pp. 232–241., doi:10.1016/j.solmat.2016.01.033.
- Lazdovica, K., et al. “Catalytic pyrolysis of wheat bran for hydrocarbons production in the presence of zeolites and noble-Metals by using TGA-FTIR method.” Bioresource Technology, vol. 207, 2016, pp. 126–133., doi:10.1016/j.biortech.2016.01.117.
- Gignone, Andrea. “Ordered Mesoporous Silica for Drug Delivery in Topical Applications.” Tesi di dottorato, 2016, p. 136., doi:10.6092/polito/porto/2652565.
- Rios, Albertina Gonçalves. “Chromatographic Separation of Dyes in a Fixed Bed Adsorber.” LSRE – Laboratory of Separation and Reaction Engineering, 2017.
- Mazzeo, Nicolas. "Silici mesoporose come carrier di idrocortisone= Mesoporous silicas as hydrortisone carriers." PhD diss., Politecnico di Torino, 2018.
- Mazzeo, Nicolas. "Silici mesoporose come carrier di idrocortisone= Mesoporous silicas as hydrortisone carriers." PhD diss., Politecnico di Torino, 2018.
- Dumitriu, Georgiana-Diana, Nieves López de Lerma, Valeriu V. Cotea, and Rafael A. Peinado. "Application of Mesoporous Materials as Fining Agents for Pedro Ximénez Wines." (2018).
- El Roz, Ayman, Pascal Fongarland, and Mickael Capron. "Glycerol to Glyceraldehyde Oxidation Reaction Over Pt-Based Catalysts Under Base-Free Conditions." Frontiers in Chemistry 7 (2019): 156.
|
-
FAQ
1. What is the collapsing temperature of MCM-48? ·
About 750 degrees Celsius.
Research Citations of ACS Material Products
- Park, Sunghwan, et al. “3-Dimensionally disordered mesoporous silica (DMS)-Containing mixed matrix membranes for CO2 and non-CO2 greenhouse gas separations.” Separation and Purification Technology, vol. 136, 2014, pp. 286–295., doi:10.1016/j.seppur.2014.09.016.
- Martín, C.f., et al. “Wet impregnation of a commercial low cost silica using DETA for a fast post-Combustion CO 2 capture process.” Applied Energy, vol. 183, 2016, pp. 1705–1721., doi:10.1016/j.apenergy.2016.09.081.
|
-
FAQ
1. What is the thickness of the SiO2 layer of Mechanically Exfoliated Monolayer MoS2 on SiO2 Substrates? Is this a natively oxidized layer or a thermally oxidized layer?
The thickness of SiO2 layer is about 300nm, and it’s a thermally oxidized layer.
|
-
FAQ
1. What is the source material of this product?
Natural graphite is the source material of our Mechanically Exfoliated Single Crystal Graphene on SiO2/Si.
|
-
FAQ
1. What is "loss on ignition" with regards to 13X?
"Loss on ignition" describes the weight change of a sample after it has been heated to high temperature causing some of its content to burn. It helps determine whether the raw material needs to be calcined in advance when it is used, so that the volume of raw material is stable.
|
-
FAQ
1.What is the specific particle size of the Molybdenum Disulfide (MoS2) Powder?
The particle size of the Molybdenum Disulfide (MoS2) Powder is 0.7±0.15 μm.
Research Citations of ACS Material Products
- Lu, Chang, et al. “Comparison of MoS2, WS2, and Graphene Oxide for DNA Adsorption and Sensing.” Langmuir, vol. 33, no. 2, May 2017, pp. 630–637., doi:10.1021/acs.langmuir.6b04502.
- Liu, Yibo, and Juewen Liu. “Hybrid nanomaterials of WS2 or MoS2 nanosheets with liposomes: biointerfaces and multiplexed drug delivery.” Nanoscale, vol. 9, no. 35, 2017, pp. 13187–13194.
- Wang, Liu, et al. “Transition Metal Dichalcogenide Nanosheets for Visual Monitoring PCR Rivaling a Real-Time PCR Instrument.” ACS Applied Materials & Interfaces, vol. 10, no. 5, 2018, pp. 4409–4418., doi:10.1021/acsami.7b15746.
- Giust, Davide, María Isabel Lucío, Otto L. Muskens, and Antonios G. Kanaras. "Interactions of DNA coated upconversion nanoparticles with 2D materials." In Colloidal Nanoparticles for Biomedical Applications XIII, vol. 10507, p. 105070U. International Society for Optics and Photonics, 2018.
- Lau, K. Y., A. A. Latif, MH Abu Bakar, F. D. Muhammad, M. F. Omar, and M. A. Mahdi. "Mechanically deposited tungsten disulfide saturable absorber for low-threshold Q-switched erbium-doped fiber laser." Applied Physics B 123, no. 8 (2017): 221.
- Hejazi, Davoud, Shuangjun Liu, Sarah Ostadabbas, and Swastik Kar. "Bayesian Inference-enabled Precise Optical Wavelength Estimation using Transition Metal Dichalcogenide Thin Films." arXiv preprint arXiv:1901.09452 (2019).
|
-
FAQ
1. What template is used in MSNS synthesis process to create the mesopores?
The template used in the synthesis is CTAB.
Research Citations of ACS Material Products
- Mortera, Renato Silvio. COMPOSITIONS FOR THE TREATMENT OF MUCOUS MEMBRANE DISEASES. 11 Dec. 2014.
|
-
FAQ
1. Is it necessary to remove LiOH before filtering MoS2 on the support membrane?
There is a tiny amount of LiOH in the solution. LiOH is soluble in the solution so it will be filtered with the solution when you filter the MoS2 nanosheets using the membrane. There’s no need to remove LiOH before using it on the support membrane.
2. What is the ratio of 2H/1T phases of monolayer MoS2 powder?
About 60%.
3. What is the production process of conductive monolayer MoS2 flakes?
MoS2 reacts with n-Butyllithium to generate LiXMoS2. Li is between the MoS2 layers. The hydrogen produced by the reaction of Li and H2O can expand MoS2. In other words, LIXMoS2 reacts with water to directly generate MoS2 flakes.
4. How can I ultrasonically disperse monolayer MoS2 powder products in water?
If the cold-dried powder is re-dispersed, the dispersion stability will not be very good due to its low oxygen content. It is recommended that the concentration does not exceed 0.1 mg/mL during dispersion. Generally, 30 minutes of ultrasonication of more than 300 watts is sufficient.
Research Citations of ACS Material Products
- Lu, Chang, et al. “Comparison of MoS2, WS2, and Graphene Oxide for DNA Adsorption and Sensing.” Langmuir, vol. 33, no. 2, May 2017, pp. 630–637., doi:10.1021/acs.langmuir.6b04502.
- Wang, Liu, et al. “Transition Metal Dichalcogenide Nanosheets for Visual Monitoring PCR Rivaling a Real-Time PCR Instrument.” ACS Applied Materials & Interfaces, vol. 10, no. 5, 2018, pp. 4409–4418., doi:10.1021/acsami.7b15746.
- Giust, Davide, et al. "Interactions of DNA coated upconversion nanoparticles with 2D materials." Colloidal Nanoparticles for Biomedical Applications XIII. Vol. 10507. International Society for Optics and Photonics, 2018.
- Lau, K. Y., A. A. Latif, MH Abu Bakar, F. D. Muhammad, M. F. Omar, and M. A. Mahdi. "Mechanically deposited tungsten disulfide saturable absorber for low-threshold Q-switched erbium-doped fiber laser." Applied Physics B 123, no. 8 (2017): 221.
- Giust, Davide, María Isabel Lucío, Otto L. Muskens, and Antonios G. Kanaras. "Interactions of DNA coated upconversion nanoparticles with 2D materials." In Colloidal Nanoparticles for Biomedical Applications XIII, vol. 10507, p. 105070U. International Society for Optics and Photonics, 2018.
- Liu, Yibo, and Juewen Liu. "Hybrid nanomaterials of WS 2 or MoS 2 nanosheets with liposomes: biointerfaces and multiplexed drug delivery." Nanoscale 9, no. 35 (2017): 13187-13194.
- Giust, Davide, María Isabel Lucío, Otto L. Muskens, and Antonios G. Kanaras. "Interactions of DNA coated upconversion nanoparticles with 2D materials." In Colloidal Nanoparticles for Biomedical Applications XIII, vol. 10507, p. 105070U. International Society for Optics and Photonics, 2018.
|
-
Research Citations of ACS Material Products
- Lu, Chang, et al. “Comparison of MoS2, WS2, and Graphene Oxide for DNA Adsorption and Sensing.” Langmuir, vol. 33, no. 2, May 2017, pp. 630–637., doi:10.1021/acs.langmuir.6b04502.
- Wang, Liu, et al. “Transition Metal Dichalcogenide Nanosheets for Visual Monitoring PCR Rivaling a Real-Time PCR Instrument.” ACS Applied Materials & Interfaces, vol. 10, no. 5, 2018, pp. 4409–4418., doi:10.1021/acsami.7b15746.
- Giust, Davide, et al. "Interactions of DNA coated upconversion nanoparticles with 2D materials." Colloidal Nanoparticles for Biomedical Applications XIII. Vol. 10507. International Society for Optics and Photonics, 2018.
- Lau, K. Y., A. A. Latif, MH Abu Bakar, F. D. Muhammad, M. F. Omar, and M. A. Mahdi. "Mechanically deposited tungsten disulfide saturable absorber for low-threshold Q-switched erbium-doped fiber laser." Applied Physics B 123, no. 8 (2017): 221.
- Liu, Yibo, and Juewen Liu. "Hybrid nanomaterials of WS 2 or MoS 2 nanosheets with liposomes: biointerfaces and multiplexed drug delivery." Nanoscale 9, no. 35 (2017): 13187-13194.
- Giust, Davide, María Isabel Lucío, Otto L. Muskens, and Antonios G. Kanaras. "Interactions of DNA coated upconversion nanoparticles with 2D materials." In Colloidal Nanoparticles for Biomedical Applications XIII, vol. 10507, p. 105070U. International Society for Optics and Photonics, 2018.
|
-
FAQ
1. What are the form of these two products?
Mordenite zeolite Type A and Type B are H-form.
|
-
FAQ
1.What is the density and diameter of Nano H-ZSM-5?
The Nano H-ZSM-5 has a diameter of 0.55nm-0.60nm with a density of about 121.6g/cm3.
Research Citations of ACS Material Products
- Devrim, Yılser, and Ayhan Albostan. “Enhancement of PEM fuel cell performance at higher temperatures and lower humidities by high performance membrane electrode assembly based on Nafion/Zeolite membrane.” International Journal of Hydrogen Energy, vol. 40, no. 44, 2015, pp. 15328–15335., doi:10.1016/j.ijhydene.2015.02.078.
- Al Naimi, Essa I., and Arthur A. Garforth. "The Effect of Zeolite Structure and Pore Systems on Maximizing Propylene Production in FCC Unit." CHEMICAL ENGINEERING 43 (2015).
- Arellano, Ian Harvey, et al. “High capacity CO2 sorbents based on zinc-Functionalized ionic liquid confined in morphologically diverse porous matrices.” RSC Advances, vol. 5, no. 80, 2015, pp. 65074–65083., doi:10.1039/c5ra12738f.
- Bianchini, Emma, et al. “Pyrolysis of spirulina and zeolite cracking over HZSM-5. An analytical investigation on the chemical route of bio-Oil from cultivation to combustion.” Journal of Analytical and Applied Pyrolysis, vol. 126, 2017, pp. 230–238., doi:10.1016/j.jaap.2017.06.004.
- Pinho, Bruno, et al. “Role of HZSM-5 Aluminosilicates on Asphaltenes Deposition by High-Throughput in Situ Characterizations of a Microreservoir.” Energy & Fuels, vol. 31, no. 11, 2017, pp. 11640–11650., doi:10.1021/acs.energyfuels.7b01748.
- Madani, S. Hadi, et al. “Particle and cluster analyses of silica powders via small angle neutron scattering.” Powder Technology, vol. 327, 2018, pp. 96–108., doi:10.1016/j.powtec.2017.12.061.
- Lu, Limin, and John T.W. Yeow. “An adsorption study of indoxyl sulfate by zeolites and polyethersulfone–zeolite composite membranes.” Materials & Design, vol. 120, 2017, pp. 328–335., doi:10.1016/j.matdes.2017.01.094.
- Sarwar, Saad, et al. “Improved Long-Term Stability of Dye-Sensitized Solar Cell by Zeolite Additive in Electrolyte.” Electrochimica Acta, vol. 245, 2017, pp. 526–530., doi:10.1016/j.electacta.2017.05.191.
- Lu, Limin, et al. “Influence of zeolite shape and particle size on their capacity to adsorb uremic toxin as powders and as fillers in membranes.” Journal of Biomedical Materials Research Part B: Applied Biomaterials, vol. 105, no. 6, 2016, pp. 1594–1601., doi:10.1002/jbm.b.33698.
- Malyala, Rajashekharam, et al. “CROSS-REFERENCE(S) TO RELATED APPLICATIONS.” BIOFUEL PRODUCTION USING NANOZEOLITE CATALYST - Cool Planet Energy Systems, Inc., 12 Jan. 2017.
- Chen, Weiqi, Priyangi Vashistha, Andrew Yen, Nikhil Joshi, Yogesh Kapoor, and Ryan L. Hartman. "Asphaltenes Dissolution Mechanism Study by in Situ Raman Characterization of a Packed-Bed Microreactor with HZSM-5 Aluminosilicates." Energy & Fuels 32, no. 12 (2018): 12205-12217.
- Ledwoch, Daniela, Emma Kendrick, and Paul Adamson. "Composite electrode including microporous ionically conducting material, composite slurry, and methods of manufacturing same." U.S. Patent Application 15/475,701, filed October 4, 2018.
- Lai, Qianxi. "Gas sensor element." U.S. Patent Application 15/576,155, filed May 17, 2018.
|
-
Research Citations of ACS Material Products
- Lu, Chang, et al. “Comparison of MoS2, WS2, and Graphene Oxide for DNA Adsorption and Sensing.” Langmuir, vol. 33, no. 2, May 2017, pp. 630–637., doi:10.1021/acs.langmuir.6b04502.
- Wang, Liu, et al. “Transition Metal Dichalcogenide Nanosheets for Visual Monitoring PCR Rivaling a Real-Time PCR Instrument.” ACS Applied Materials & Interfaces, vol. 10, no. 5, 2018, pp. 4409–4418., doi:10.1021/acsami.7b15746.
- Giust, Davide, María Isabel Lucío, Otto L. Muskens, and Antonios G. Kanaras. "Interactions of DNA coated upconversion nanoparticles with 2D materials." In Colloidal Nanoparticles for Biomedical Applications XIII, vol. 10507, p. 105070U. International Society for Optics and Photonics, 2018.
- Lau, K. Y., A. A. Latif, MH Abu Bakar, F. D. Muhammad, M. F. Omar, and M. A. Mahdi. "Mechanically deposited tungsten disulfide saturable absorber for low-threshold Q-switched erbium-doped fiber laser." Applied Physics B 123, no. 8 (2017): 221.
- Liu, Yibo, and Juewen Liu. "Hybrid nanomaterials of WS 2 or MoS 2 nanosheets with liposomes: biointerfaces and multiplexed drug delivery." Nanoscale 9, no. 35 (2017): 13187-13194.
|
-
FAQ
1. What’s the difference between Nano Size Monolayer WS2 and Monolayer WS2?
Nano Size Monolayer WS2 is prepared by lithium-based intercalation method, and Monolayer WS2 is prepared by dry method.
Research Citations of ACS Material Products
- Lu, Chang, et al. “Comparison of MoS2, WS2, and Graphene Oxide for DNA Adsorption and Sensing.” Langmuir, vol. 33, no. 2, May 2017, pp. 630–637., doi:10.1021/acs.langmuir.6b04502.
- Wang, Liu, et al. “Transition Metal Dichalcogenide Nanosheets for Visual Monitoring PCR Rivaling a Real-Time PCR Instrument.” ACS Applied Materials & Interfaces, vol. 10, no. 5, 2018, pp. 4409–4418., doi:10.1021/acsami.7b15746.
- Giust, Davide, María Isabel Lucío, Otto L. Muskens, and Antonios G. Kanaras. "Interactions of DNA coated upconversion nanoparticles with 2D materials." In Colloidal Nanoparticles for Biomedical Applications XIII, vol. 10507, p. 105070U. International Society for Optics and Photonics, 2018.
- Lau, K. Y., A. A. Latif, MH Abu Bakar, F. D. Muhammad, M. F. Omar, and M. A. Mahdi. "Mechanically deposited tungsten disulfide saturable absorber for low-threshold Q-switched erbium-doped fiber laser." Applied Physics B 123, no. 8 (2017): 221.
- Liu, Yibo, and Juewen Liu. "Hybrid nanomaterials of WS 2 or MoS 2 nanosheets with liposomes: biointerfaces and multiplexed drug delivery." Nanoscale 9, no. 35 (2017): 13187-13194.
|
-
Research Citations of ACS Material Products
- Dasgupta, Subho, et al. “Toward On-and-Off Magnetism: Reversible Electrochemistry to Control Magnetic Phase Transitions in Spinel Ferrites.” Advanced Functional Materials, vol. 26, no. 41, July 2016, pp. 7507–7515., doi:10.1002/adfm.201603411.
- Nguyen, Trung Kien, et al. “Experimental Results on Lamellar-Type Solid Lubricants in Enhancing Minimum Quantity Lubrication Machining.” Journal of Manufacturing Science and Engineering, vol. 138, no. 10, 2016, p. 101011., doi:10.1115/1.4033995.
- Sakthivel, Mariappan, and Jean-Francois Drillet. "An extensive study about influence of the carbon support morphology on Pt activity and stability for oxygen reduction reaction." Applied Catalysis B: Environmental 231 (2018): 62-72.
|
-
Research Citations of ACS Material Products
- Zhang, Zimeng, et al. “Roll-to-Roll printing of flexible thin-Film organic thermoelectric devices.” Manufacturing Letters, vol. 8, 2016, pp. 6–10., doi:10.1016/j.mfglet.2016.04.002.
- Nieto, Andy, et al. “Graphene reinforced metal and ceramic matrix composites: a review.” International Materials Reviews, vol. 62, no. 5, 2016, pp. 241–302., doi:10.1080/09506608.2016.1219481.
- Deng, Wenfang, et al. “Three-Dimensional nitrogen-Doped graphene derived from poly-o-Phenylenediamine for high-Performance supercapacitors.” Journal of Electroanalytical Chemistry, vol. 787, 2017, pp. 103–109., doi:10.1016/j.jelechem.2017.01.047.
- Glebova, Yulia, et al. “Nitrogen-Doped graphene as an alternative to ecotoxic zinc oxide in rubbers.” Journal of Applied Polymer Science, vol. 135, no. 17, Oct. 2018, p. 46116., doi:10.1002/app.46116.
- Meng, Xiangbo, et al. “Atomic Layer Deposition of Aluminum Sulfide: Growth Mechanism and Electrochemical Evaluation in Lithium-Ion Batteries.” Chemistry of Materials, vol. 29, no. 21, 2017, pp. 9043–9052., doi:10.1021/acs.chemmater.7b02175.
- Sun, Qian. "Interfacial Tailoring of Lithium-ion Batteries by Atomic/Molecular Layer Deposition." (2018).
- Yang, Yiqun, Kayla Strong, Gaind P. Pandey, and Lamartine Meda. "Nanostructured V 2 O 5/Nitrogen-doped Graphene Hybrids for High Rate Lithium Storage." MRS Advances 3, no. 60 (2018): 3495-3500.
|
-
FAQ
1. Which precursor was used for the preparation?
Sucrose was used as the precursor for CMK-3.
2. What was the applied heat-treatment temperature for the carbonization?
Carbonization was treated at 900°C for 5h under an inert atmosphere of nitrogen.
Research Citations of ACS Material Products
- Minella, Christian Bonatto, et al. “NaAlH4 confined in ordered mesoporous carbon.” International Journal of Hydrogen Energy, vol. 38, no. 21, 2013, pp. 8829–8837., doi:10.1016/j.ijhydene.2013.04.103.
- Zhang, Junshe, and Jae W. Lee. “Supercapacitor Electrodes Derived from Carbon Dioxide.” ACS Sustainable Chem. Eng., 20 Dec. 2013, pp. 735–740., doi:10.1021/sc400414r.
- Carrales-Alvarado, D.H., et al. “Removal of the antibiotic metronidazole by adsorption on various carbon materials from aqueous phase.” Journal of Colloid and Interface Science, vol. 436, 2014, pp. 276–285., doi:10.1016/j.jcis.2014.08.023.
- Zalupski, Peter R., et al. “The Adsorption of Gold, Palladium, and Platinum from Acidic Chloride Solutions on Mesoporous Carbons.” Solvent Extraction and Ion Exchange, vol. 32, no. 7, Oct. 2014, pp. 737–748., doi:10.1080/07366299.2014.951278.
- Hark, E., et al. “Different Carbide Derived Nanoporous Carbon Supports and Electroreduction of Oxygen.” ECS Transactions, vol. 66, no. 24, Nov. 2015, pp. 69–80., doi:10.1149/06624.0069ecst.
- Roznyatovskaya, Nataliya, et al. “Sieving Effects in Electrical Double-Layer Capacitors Based on Neat [Al(Hfip)4]−and [NTf2]−Ionic Liquids.” ChemElectroChem, vol. 2, no. 6, June 2015, pp. 829–836., doi:10.1002/celc.201500024.
- Kim, Jae Ho. “Stabilization of Insoluble Discharge Products by Facile Aniline Modification for High Performance Li-S Batteries.” Advanced Energy Materials, vol. 5, no. 14, 15 May 2015, doi:10.1002/aenm.201500268.
- Salameh, Chrystelle, et al. “Monodisperse platinum nanoparticles supported on highly ordered mesoporous silicon nitride nanoblocks: superior catalytic activity for hydrogen generation from sodium borohydride.” RSC Advances, vol. 5, no. 72, 2015, pp. 58943–58951., doi:10.1039/c5ra05901a.
- Mercier, Guillaume, et al. “Porous Graphene Oxide/Diboronic Acid Materials: Structure and Hydrogen Sorption.” The Journal of Physical Chemistry C, vol. 119, no. 49, 2015, pp. 27179–27191., doi:10.1021/acs.jpcc.5b06402.
- Walter, Marc. “Efficient and Inexpensive Sodium–Magnesium Hybrid Battery.” Chemistry of Materials, 16 Oct. 2015, pp. 7452–7458., doi:10.1021/acs.chemmater.5b03531.
- Shih, Yung-Han, et al. “Nanoporous Carbons Derived from Metal-Organic Frameworks as Novel Matrices for Surface-Assisted Laser Desorption/Ionization Mass Spectrometry.” Small, vol. 12, no. 15, 23 Feb. 2016, doi:10.1002/smll.201502817.
- Yoo, Eunjoo, and Haoshen Zhou. “Carbon Cathodes in Rechargeable Lithium-Oxygen Batteries Based on Double-Lithium-Salt Electrolytes.” ChemSusChem, vol. 9, no. 11, 2016, pp. 1249–1254., doi:10.1002/cssc.201600177.
- Pięk, Magdalena, et al. “The Complex Crystal of NaTCNQ–TCNQ Supported on Different Carbon Materials as Ion-to-Electron Transducer in All-Solid-State Sodium-Selective Electrode.” Journal of The Electrochemical Society, vol. 163, no. 13, 2016, doi:10.1149/2.0341613jes.
- Yin, Wang, et al. “A One-Step Synthesis of C6 Sugar Alcohols from Levoglucosan and Disaccharides Using a Ru/CMK-3 Catalyst.” ACS Catalysis, vol. 6, no. 7, 2016, pp. 4411–4422., doi:10.1021/acscatal.6b00296.
- See, Kimberly A., et al. “Effect of Hydrofluoroether Cosolvent Addition on Li Solvation in Acetonitrile-Based Solvate Electrolytes and Its Influence on S Reduction in a Li–S Battery.” ACS Applied Materials & Interfaces, vol. 8, no. 50, Aug. 2016, pp. 34360–34371., doi:10.1021/acsami.6b11358.
- Mi, Yingying, et al. “A pomegranate-Structured sulfur cathode material with triple confinement of lithium polysulfides for high-Performance lithium–sulfur batteries.” Journal of Materials Chemistry A, vol. 5, no. 23, 2017, pp. 11788–11793., doi:10.1039/c7ta00035a.
- Shih, Yung-Han, et al. “Nitrogen-Doped porous carbon material derived from metal–organic gel for small biomolecular sensing.” Chemical Communications, vol. 53, no. 42, 2017, pp. 5725–5728., doi:10.1039/c7cc00665a.
- Shin, Minjeong, et al. “Effect of the Hydrofluoroether Cosolvent Structure in Acetonitrile-Based Solvate Electrolytes on the Li Solvation Structure and Li–S Battery Performance.” ACS Applied Materials & Interfaces, vol. 9, no. 45, 2017, pp. 39357–39370., doi:10.1021/acsami.7b11566.
- Kwon, Ji Eon, et al. “Triptycene-Based quinone molecules showing multi-Electron redox reactions for large capacity and high energy organic cathode materials in Li-Ion batteries.” Journal of Materials Chemistry A, 2018, doi:10.1039/c7ta09968a.
- Chen, Zhihua, et al. “Development of a reactor with carbon catalysts for modular-Scale, low-Cost electrochemical generation of H2O2.” Reaction Chemistry & Engineering, vol. 2, no. 2, 2017, pp. 239–245., doi:10.1039/c6re00195e.
- Jumaah, Alaa, Shihab Al-Daffaie, Oktay Yilmazoglu, and Franko Küppers. "Graphene Enhanced 2-D Nanoelectrode for Continuous Wave Terahertz Photomixers." In 2018 43rd International Conference on Infrared, Millimeter, and Terahertz Waves (IRMMW-THz), pp. 1-2. IEEE, 2018.
- Kim, Minjae, Eunjoo Yoo, Wha-Seung Ahn, and Sang Eun Shim. "Controlling porosity of porous carbon cathode for lithium oxygen batteries: Influence of micro and meso porosity." Journal of Power Sources 389 (2018): 20-27.
- Sakthivel, Mariappan, and Jean-Francois Drillet. "An extensive study about influence of the carbon support morphology on Pt activity and stability for oxygen reduction reaction." Applied Catalysis B: Environmental 231 (2018): 62-72.
- Li, Lu, Lijuan Hou, Jie Cheng, Trevor Simmons, Fuming Zhang, Lucy T. Zhang, Robert J. Linhardt, and Nikhil Koratkar. "A flexible carbon/sulfur-cellulose core-shell structure for advanced lithium–sulfur batteries." Energy Storage Materials 15 (2018): 388-395.
- Kim, Minjae, Eunjoo Yoo, Wha-Seung Ahn, and Sang Eun Shim. "Controlling porosity of porous carbon cathode for lithium oxygen batteries: Influence of micro and meso porosity." Journal of Power Sources 389 (2018): 20-27.
- Min, Dong Joo, Fabien Miomandre, Pierre Audebert, Ji Eon Kwon, and Soo Young Park. "s‐Tetrazines as a New Electrode‐Active Material for Secondary Batteries." ChemSusChem 12, no. 2 (2019): 503-510.
- Kaldenhoven, Richard G., and Josephine M. Hill. "Determining the pore structure of activated carbon by nitrogen gas adsorption." In Catalysis, vol. 30, pp. 41-63. The Royal Society of Chemistry, 2018.
- Fan, Xiulin, Jie Yue, Fudong Han, Ji Chen, Tao Deng, Xiuquan Zhou, Singyuk Hou, and Chunsheng Wang. "High-performance all-solid-state Na–S battery enabled by casting–annealing technology." ACS nano 12, no. 4 (2018): 3360-3368.
- Pinck, Stéphane, Frédéric PA Jorand, Mengjie Xu, and Mathieu Etienne. "Protamine promotes direct electron transfer between Shewanella oneidensis cells and carbon nanomaterials in bacterial biocomposites." ChemElectroChem.
|
-
Research Citations of ACS Material Products
- Zheng, Shiyou, et al. “Batteries: Nano-Copper-Assisted Immobilization of Sulfur in High-Surface-Area Mesoporous Carbon Cathodes for Room Temperature Na-S Batteries (Adv. Energy Mater. 12/2014).” Advanced Energy Materials, vol. 4, no. 12, 2014, doi:10.1002/aenm.201470065.
- Bi, Yujing, et al. “Chemistry and Electrochemical Performance of Mg Electrolytes for Rechargeable Mg Batteries: A Study of Mg Powder Scavenger.” ECS Transactions, vol. 80, no. 10, 2017, pp. 343–348., doi:10.1149/08010.0343ecst.
- Liu, Tianbiao. "Magnesium-based electrolyte compositions and uses thereof." U.S. Patent Application 16/033,087, filed January 17, 2019.
|
-
Research Citations of ACS Material Products
1. Zheng, Shiyou, et al. “Copper-Stabilized Sulfur-Microporous Carbon Cathodes for Li-S Batteries.” Advanced Functional Materials, vol. 24, no. 26, 2014, pp. 4156–4163., doi:10.1002/adfm.201304156. 2. Saha, Dipendu, et al. “Micro-/Mesoporous carbons for controlled release of antipyrine and indomethacin.” RSC Advances, vol. 5, no. 30, 2015, pp. 23699–23707., doi:10.1039/c5ra00251f. 3. Karim, Hasanul, et al. “Porous carbon/CeO2 composites for Li-Ion battery application.” Proc. SPIE 9439, 27 Mar. 2015, doi:10.1117/12.2084293. 4. Shuvo, Mohammad Arif I., et al. “High-Performance porous carbon/CeO2nanoparticles hybrid super-Capacitors for energy storage.” Smart Materials and Nondestructive Evaluation for Energy Systems 2015, 2015, doi:10.1117/12.2084267. 5. Andalibi, Mohammad R., et al. “Evidence of Nanoconfinement Effects in the Adsorption of Hydrogen on Coinage Metal Complexes Dispersed within Porous Carbon.” The Journal of Physical Chemistry C, vol. 119, no. 37, Apr. 2015, pp. 21314–21322., doi:10.1021/acs.jpcc.5b05173. 6. Shuvo, Mohammad Arif Ishtiaque, et al. “Microwave exfoliated graphene oxide/TiO2 nanowire hybrid for high performance lithium ion battery.” Journal of Applied Physics, vol. 118, no. 12, 2015, p. 125102., doi:10.1063/1.4931380. 7. Saha, Dipendu. “A study on the cytotoxicity of carbon-Based materials.” Materials Science and Engineering: C, vol. 68, 1 Nov. 2016, doi:10.1016/j.msec.2016.05.094. 8. Alam, Todd M., and Thomas M. Osborn Popp. “In-Pore exchange and diffusion of carbonate solvent mixtures in nanoporous carbon.” Chemical Physics Letters, vol. 658, 2016, pp. 51–57., doi:10.1016/j.cplett.2016.06.014. 9. Andalibi, Mohammad R., and Henry C. Foley. “Temperature-Dependent nature of interaction between hydrogen and copper(II) acetate confined in nanoporous carbon.” International Journal of Hydrogen Energy, vol. 41, no. 20, 2016, pp. 8506–8513., doi:10.1016/j.ijhydene.2016.03.044. 10. Saha, Dipendu, et al. “Characteristics of Methane Adsorption in Micro-Mesoporous Carbons at Low and Ultra-High Pressure.” Energy Technology, vol. 4, no. 11, 2016, pp. 1392–1400., doi:10.1002/ente.201600172. 11. Wang, Xiwen, et al. “Tailoring Surface Acidity of Metal Oxide for Better Polysulfide Entrapment in Li-S Batteries.” Advanced Functional Materials, vol. 26, no. 39, Mar. 2016, pp. 7164–7169., doi:10.1002/adfm.201602264. 12. Kim, Hoejin, et al. “Synthesis and characterization of CeO2 nanoparticles on porous carbon for Li-Ion battery.” MRS Advances, vol. 2, no. 54, 2017, pp. 3299–3307., doi:10.1557/adv.2017.443. 13. Daubert, James S., et al. “Intrinsic limitations of atomic layer deposition for pseudocapacitive metal oxides in porous electrochemical capacitor electrodes.” Journal of Materials Chemistry A, vol. 5, no. 25, 2017, pp. 13086–13097., doi:10.1039/c7ta02719b. 14. Ju, Jaechul, et al. “3D in-Situ hollow carbon fiber/Carbon nanosheet/Fe 3 C@Fe 3 O 4 by solventless one-Step synthesis and its superior supercapacitor performance.” Electrochimica Acta, vol. 252, 2017, pp. 215–225., doi:10.1016/j.electacta.2017.09.002. 15. Zhao, Changtai, et al. “Enhanced sodium storage capability enabled by super wide-Interlayer-Spacing MoS 2 integrated on carbon fibers.” Nano Energy, vol. 41, 2017, pp. 66–74., doi:10.1016/j.nanoen.2017.08.030. 16. Hasyim, Muhammad R., et al. “Prediction of Charge-Discharge and Impedance Characteristics of Electric Double-Layer Capacitors Using Porous Electrode Theory.” Journal of The Electrochemical Society, vol. 164, no. 13, 2017, doi:10.1149/2.0051713jes. 17. Chen, Changjiu, et al. “Higher-Order glass-Transition singularities in nano-Confined states.” RSC Adv., vol. 7, no. 75, 2017, pp. 47801–47805., doi:10.1039/c7ra09049h. 18. Karim, Hasanul, et al. “Feasibility study of thermal energy harvesting using lead free pyroelectrics.” Smart Materials and Structures, vol. 25, no. 5, May 2016, p. 055022., doi:10.1088/0964-1726/25/5/055022. 19. Yang, Yuanying, Weiqin Wang, Yanna Nuli, Jun Yang, and Jiulin Wang. "High Active Magnesium Trifluoromethanesulfonate-Based Electrolytes for Magnesium-Sulfur Batteries." ACS applied materials & interfaces (2019). 20. Wang, Weiqin, Hancheng Yuan, Yanna NuLi, Jingjing Zhou, Jun Yang, and Jiulin Wang. "Sulfur@ microporous Carbon Cathode with a High Sulfur Content for Magnesium–Sulfur Batteries with Nucleophilic Electrolytes." The Journal of Physical Chemistry C 122, no. 46 (2018): 26764-26776.
|
-
FAQ
1. Can Pyrolytic Graphite Powder be suspended in an epoxy or urethane and poured into a shape?
Yes.
2. Is Pyrolytic Graphite highly diamagnetic?
Pyrolytic Graphite powder is a kind of weak magnetic material, and it’s regarded as a material with relatively high diamagnetism among weak magnetic materials.
Research Citations of ACS Material Products
1. Ellingsen, Linda, Alex Holland, Jean-Francois Drillet, Willi Peters, Martin Eckert, Carlos Concepcion, Oscar Ruiz et al. "Environmental screening of electrode materials for a rechargeable aluminum battery with an AlCl3/EMIMCl electrolyte." Materials 11, no. 6 (2018): 936.
|
-
Research Citations of ACS Material Products
1. Vicentini, Nicola, Teresa Gatti, Marco Salerno, Yuriko Suemi Hernandez Gomez, Mattia Bellon, Sasha Gallio, Carla Marega, Francesco Filippini, and Enzo Menna. "Effect of different functionalized carbon nanostructures as fillers on the physical properties of biocompatible poly (l-lactic acid) composites." Materials Chemistry and Physics 214 (2018): 265-276.
|
-
FAQ
Is SAPO-11 H+ or Na+ form?
SAPO-11 is H+ form.
Research Citations of ACS Material Products
- Verboekend, Danny, et al. “Hierarchical Silicoaluminophosphates by Postsynthetic Modification: Influence of Topology, Composition, and Silicon Distribution.” Chemistry of Materials, vol. 26, no. 15, 2014, pp. 4552–4562., doi:10.1021/cm501774s.
- Pérez, William, et al. “Upgrading of palm oil renewable diesel through hydroisomerization and formulation of an optimal blend.” Fuel, vol. 209, 2017, pp. 442–448., doi:10.1016/j.fuel.2017.08.013.
- Zhao, Pu, Lin Ye, Zhenyu Sun, Benedict TW Lo, Harry Woodcock, Chen Huang, Chiu Tang, Angus I. Kirkland, Donghai Mei, and Shik Chi Edman Tsang. "Entrapped single tungstate site in zeolite for cooperative catalysis of olefin metathesis with Brønsted acid site." Journal of the American Chemical Society 140, no. 21 (2018): 6661-6667.
|
-
FAQ
What is the calcination temperature of SAPO-34?
It is 550 Celsius.
Research Citations of ACS Material Products
- Wang, Di, et al. “NH3-SCR over Cu/SAPO-34 – Zeolite acidity and Cu structure changes as a function of Cu loading.” Catalysis Today, vol. 231, 2014, pp. 64–74., doi:10.1016/j.cattod.2013.11.040.
- Verboekend, Danny, et al. “Hierarchical Silicoaluminophosphates by Postsynthetic Modification: Influence of Topology, Composition, and Silicon Distribution.” Chemistry of Materials, vol. 26, no. 15, 2014, pp. 4552–4562., doi:10.1021/cm501774s.
- Gao, Feng, et al. “Synthesis and evaluation of Cu/SAPO-34 catalysts for NH 3 -SCR 2: Solid-State ion exchange and one-Pot synthesis.” Applied Catalysis B: Environmental, vol. 162, 2015, pp. 501–514., doi:10.1016/j.apcatb.2014.07.029.
- Wang, Di, et al. “A comparison of hydrothermal aging effects on NH3-SCR of NOx over Cu-SSZ-13 and Cu-SAPO-34 catalysts.” Applied Catalysis B: Environmental, vol. 165, 2015, pp. 438–445., doi:10.1016/j.apcatb.2014.10.020.
- Andonova, Stanislava, et al. “The effect of iron loading and hydrothermal aging on one-Pot synthesized Fe/SAPO-34 for ammonia SCR.” Applied Catalysis B: Environmental, vol. 180, 2016, pp. 775–787., doi:10.1016/j.apcatb.2015.07.007.
- Ahmad, N. N. R., et al. “Effect of different organic amino cations on SAPO-34 for PES/SAPO-34 mixed matrix membranes toward CO2/CH4 separation.” Journal of Applied Polymer Science, vol. 133, no. 18, 18 Jan. 2016, doi:10.1002/app.43387.
- Simonetti, Marco, et al. “Experimental testing of the buoyant functioning of a coil coated with SAPO34 zeolite, designed for solar DEC (Desiccant Evaporative Cooling) systems of buildings with natural ventilation.” Applied Thermal Engineering, vol. 103, 2016, pp. 781–789., doi:10.1016/j.applthermaleng.2016.02.072.
- Bruce, Spencer M., et al. “Small pore zeolite catalysts for furfural synthesis from xylose and switchgrass in a γ-Valerolactone/Water solvent.” Journal of Molecular Catalysis A: Chemical, vol. 422, 2016, pp. 18–22., doi:10.1016/j.molcata.2016.02.025.
- Youssef, Peter G., et al. “Numerical simulation of combined adsorption desalination and cooling cycles with integrated evaporator/Condenser.” Desalination, vol. 392, 2016, pp. 14–24., doi:10.1016/j.desal.2016.04.011.
- Van Der Perre, Stijn, et al. “Intensified Biobutanol Recovery by using Zeolites with Complementary Selectivity.” ChemSusChem, vol. 10, no. 14, May 2017, pp. 2968–2977., doi:10.1002/cssc.201700667.
- Mohshim, Dzeti Farhah, et al. “A study on carbon dioxide removal by blending the ionic liquid in membrane synthesis.” Separation and Purification Technology, 2017, doi:10.1016/j.seppur.2017.06.034.
- Borodina, E., et al. “Influence of the Reaction Temperature on the Nature of the Active and Deactivating Species during Methanol to Olefins Conversion over H-SSZ-13.” ACS Catalysis, vol. 5, no. 2, Dec. 2015, pp. 992–1003., doi:10.1021/cs501345g.
- Ghosh, Ashim Kumar, et al. SILICOALUMINOPHOSPHATE CATALYST FOR CHLOROMETHANE CONVERSION.
- Ghosh, Ashim Kumar, et al. Stable silicoaluminophosphate catalysts for conversion of alkyl halides to olefins.
- Woo, Jungwon, Kirsten Leistner, Diana Bernin, Homayoun Ahari, Mark Shost, Michael Zammit, and Louise Olsson. "Effect of various structure directing agents (SDAs) on low-temperature deactivation of Cu/SAPO-34 during NH3-SCR reaction." Catalysis Science & Technology (2018).
- Nasir, Rizwan, Nor Naimah Rosyadah Ahmad, Hilmi Mukhtar, and Dzeti Farhah Mohshim. "Effect of ionic liquid inclusion and amino–functionalized SAPO-34 on the performance of mixed matrix membranes for CO2/CH4 separation." Journal of Environmental Chemical Engineering 6, no. 2 (2018): 2363-2368.
- Zhao, Pu, Lin Ye, Zhenyu Sun, Benedict TW Lo, Harry Woodcock, Chen Huang, Chiu Tang, Angus I. Kirkland, Donghai Mei, and Shik Chi Edman Tsang. "Entrapped single tungstate site in zeolite for cooperative catalysis of olefin metathesis with Brønsted acid site." Journal of the American Chemical Society 140, no. 21 (2018): 6661-6667.
- Romo, Joelle E., Ting Wu, Xinlei Huang, Jolie Lucero, Jennifer L. Irwin, Jesse Q. Bond, Moises A. Carreon, and Stephanie G. Wettstein. "SAPO-34/5A Zeolite Bead Catalysts for Furan Production from Xylose and Glucose." ACS Omega 3, no. 11 (2018): 16253-16259.
- Fickel, Dustin, Neeta Kulkarni, and Kaiwalya Sabnis. "Synthesis of sapo-34 and use in chloromethane to olefins reactions." U.S. Patent Application 16/076,739, filed February 14, 2019.
|
-
FAQs
1. What is the final calcination temperature used during synthesis?
The material is calcined in air at 823 Kelvin for 6 hrs to decompose the triblock co-polymer and yielding a white powder, SBA-15. This is the typical method for preparing SBA-15.
2. What is the collapsing temperature of SBA-15?
About 750 degrees Celsius.
3. What are the impurity elements in SBA-15?
The impurity is Na2O, whose content is less than 0.1%.
4. Which What method was used to measure pore volume?
We use a BET specific surface area test method. The instrument itself has a specific method for determining the pore volume.
5. Which BET method is used to calculate 1) Pore volume 2) Desorption cumulative volume of BJH pores?
We used BET test of liquid nitrogen adsorption. These two data are shown in the test results.
Research Citations of ACS Material Products
- Hong, Junghyun, and Francisco Zaera. “Interference of the Surface of the Solid on the Performance of Tethered Molecular Catalysts.” Journal of the American Chemical Society, vol. 134, no. 31, 2012, pp. 13056–13065., doi:10.1021/ja304181q.
- Wang, Huali, et al. “Support effects on hydrotreating of soybean oil over NiMo carbide catalyst.” Fuel, vol. 111, 2013, pp. 81–87., doi:10.1016/j.fuel.2013.04.066.
- Kuo, Yun-Hsuan, et al. “Concurrent Observation of Bulk and Protein Hydration Water by Spin-Label ESR under Nanoconfinement.” Langmuir, vol. 29, no. 45, 2013, pp. 13865–13872., doi:10.1021/la403002t.
- Goel, Jyoti, and Suddhasatwa Basu. “Effect of support materials on the performance of direct ethanol fuel cell anode catalyst.” International Journal of Hydrogen Energy, vol. 39, no. 28, 23 Sept. 2014, pp. 15956–15966., doi:10.1016/j.ijhydene.2014.01.203.
- Piumetti, Marco, et al. “Nanostructured ceria-Based catalysts for soot combustion: Investigations on the surface sensitivity.” Applied Catalysis B: Environmental, vol. 165, 2015, pp. 742–751., doi:10.1016/j.apcatb.2014.10.062.
- Lewandowski, Dawid, et al. “Fluorescence properties of riboflavin-Functionalized mesoporous silica SBA-15 and riboflavin solutions in presence of different metal and organic cations.” Journal of Physics and Chemistry of Solids, vol. 85, 2015, pp. 56–61., doi:10.1016/j.jpcs.2015.04.007.
- Skorupska, Ewa, et al. “NMR Study of BA/FBA Cocrystal Confined Within Mesoporous Silica Nanoparticles Employing Thermal Solid Phase Transformation.” The Journal of Physical Chemistry C, vol. 119, no. 16, Aug. 2015, pp. 8652–8661., doi:10.1021/jp5123008.
- Zandavi, Seyed Hadi, and C. A. Ward. “Nucleation and growth of condensate in nanoporous materials.” Physical Chemistry, vol. 17, no. 15, 2015, pp. 9828–9834., doi:10.1039/c5cp00471c.
- Lewandowski, Dawid, et al. “Immobilization of Zidovudine Derivatives on the SBA-15 Mesoporous Silica and Evaluation of Their Cytotoxic Activity.” Plos One, vol. 10, no. 5, May 2015, doi:10.1371/journal.pone.0126251.
- Lewandowski, Dawid, et al. “SBA-15 Mesoporous Silica Modified with Gallic Acid and Evaluation of Its Cytotoxic Activity.” Plos One, vol. 10, no. 7, July 2015, doi:10.1371/journal.pone.0132541.
- Bruzzoniti, M. C., et al. “Adsorption of bentazone herbicide onto mesoporous silica: application to environmental water purification.” Environmental Science and Pollution Research, vol. 23, no. 6, 2015, pp. 5399–5409., doi:10.1007/s11356-015-5755-1.
- Jabbari-Hichri, Amira, et al. “Effect of aluminum sulfate addition on the thermal storage performance of mesoporous SBA-15 and MCM-41 materials.” Solar Energy Materials and Solar Cells, vol. 149, 2016, pp. 232–241., doi:10.1016/j.solmat.2016.01.033.
- Gignone, Andrea. “Ordered Mesoporous Silica for Drug Delivery in Topical Applications.” Tesi di dottorato, 2016, p. 136., doi:10.6092/polito/porto/2652565.
- Melnichenko, Yuri B. “Structural Characterization of Porous Materials Using SAS.” Small-Angle Scattering from Confined and Interfacial Fluids, 2016, pp. 139–171., doi:10.1007/978-3-319-01104-2_7.
- Luo, Sheng, et al. “Confinement-Induced Supercriticality and Phase Equilibria of Hydrocarbons in Nanopores.” Langmuir, vol. 32, no. 44, 2016, pp. 11506–11513., doi:10.1021/acs.langmuir.6b03177.
- Welch, Ken, et al. “Investigation of the Antibacterial Effect of Mesoporous Magnesium Carbonate.” ACS Omega, vol. 1, no. 5, 2016, pp. 907–914., doi:10.1021/acsomega.6b00124.
- Krzyżak, A.t., and I. Habina. “Low field 1 H NMR characterization of mesoporous silica MCM-41 and SBA-15 filled with different amount of water.” Microporous and Mesoporous Materials, vol. 231, 2016, pp. 230–239., doi:10.1016/j.micromeso.2016.05.032.
- Yildiz, M., et al. “Support material variation for the MnxOy-Na2WO4/SiO2 catalyst.” Catalysis Today, vol. 228, 2014, pp. 5–14., doi:10.1016/j.cattod.2013.12.024.
- Skorupska, Ewa, et al. “Thermal Solvent-Free Method of Loading of Pharmaceutical Cocrystals into the Pores of Silica Particles: A Case of Naproxen/Picolinamide Cocrystal.” The Journal of Physical Chemistry C, vol. 120, no. 24, 2016, pp. 13169–13180., doi:10.1021/acs.jpcc.6b05302.
- Ferrer, Diana Iruretagoyena. “Layered Double Hydroxides Supported on Graphene Oxide for CO2 Adsorption.” Springer Theses (Recognizing Outstanding Ph.D. Research)., 2016, doi:10.1007/978-3-319-41276-4_5.
- Stanzione, M., et al. “Peculiarities of vanillin release from amino-Functionalized mesoporous silica embedded into biodegradable composites.” European Polymer Journal, vol. 89, 2017, pp. 88–100., doi:10.1016/j.eurpolymj.2017.01.040.
- Fiorilli, Sonia, et al. “Iron oxide inside SBA-15 modified with amino groups as reusable adsorbent for highly efficient removal of glyphosate from water.” Applied Surface Science, vol. 411, 2017, pp. 457–465., doi:10.1016/j.apsusc.2017.03.206.
- Dumitriu, Georgiana-Diana, et al. “Study of the potential use of mesoporous nanomaterials as fining agent to prevent protein haze in white wines and its impact in major volatile aroma compounds and polyols.” Food Chemistry, vol. 240, 2018, pp. 751–758., doi:10.1016/j.foodchem.2017.07.163.
- Yan, Y., et al. “Synthesis, stability and Li-Ion mobility of nanoconfined Li2B12H12.” Dalton Transactions, vol. 46, no. 37, 2017, pp. 12434–12437., doi:10.1039/c7dt02946b.
- Reiser, Sarah, and Michael Turk. “Influence of the Chemical Nature of Carrier Materials on the Dissolution Behaviour of Ibuprofen.” May 2016.
- Drobná, Helena, et al. “Analysis of Ni species formed on zeolites, mesoporous silica and alumina supports and their catalytic behavior in the dry reforming of methane.” Reaction Kinetics, Mechanisms and Catalysis, vol. 121, no. 1, Sept. 2017, pp. 255–274., doi:10.1007/s11144-017-1149-3.
- Diep, Brian. “Knowledge Bank.” Analyzing Catalyst Deactivation with a Packed Bed Reactor, The Ohio State University, 1 Dec. 2017, kb.osu.edu/dspace/handle/1811/81595.
- Madani, S. Hadi, et al. “Particle and cluster analyses of silica powders via small angle neutron scattering.” Powder Technology, vol. 327, 2018, pp. 96–108., doi:10.1016/j.powtec.2017.12.061.
- Bruzzoniti, Maria C., et al. “Polymer-Derived ceramic aerogels as sorbent materials for the removal of organic dyes from aqueous solutions.” Journal of the American Ceramic Society, vol. 101, no. 2, 2017, pp. 821–830., doi:10.1111/jace.15241.
- Zandavi, Seyed Hadi. “Vapours Adsorption on Non-Porous or Porous Solids: Zeta Adsorption Isotherm Approach.” TSpace, 1 Nov. 2015, tspace.library.utoronto.ca/handle/1807/71411.
- Dumitriu, Georgiana-Diana, Nieves López de Lerma, Valeriu V. Cotea, and Rafael A. Peinado. "Application of Mesoporous Materials as Fining Agents for Pedro Ximénez Wines." (2018).
- Weigler, Max, Martin Brodrecht, Gerd Buntkowsky, and Michael Vogel. "Reorientation of Deeply Cooled Water in Mesoporous Silica: NMR Studies of the Pore-Size Dependence." The Journal of Physical Chemistry B (2019).
- Afzal, Mohammad Suhail, Faiza Zanin, Muhammad Usman Ghori, Marta Granollers, and Enes Šupuk. "The effect of mesoporous silica impregnation on tribo-electrification characteristics of flurbiprofen." International journal of pharmaceutics 544, no. 1 (2018): 55-61.
- De la Torre Paredes, Cristina. "Nanotechnology and supramolecular chemistry in controlled release and molecular recognition proceses for biomedical applications." PhD diss., 2018.
- Iqbal, Muhammad Faisal, Satoshi Tominaka, Wenqin Peng, Toshiaki Takei, Nao Tsunoji, Tsuneji Sano, and Yusuke Ide. "Iron Aquo Complex as an Efficient and Selective Homogeneous Photocatalyst for Organic Synthetic Reactions." ChemCatChem 10, no. 20 (2018): 4509-4513.
- Bruzzoniti, Maria C., Marta Appendini, Luca Rivoira, Barbara Onida, Massimo Del Bubba, Prasanta Jana, and Gian Domenico Sorarù. "Polymer‐derived ceramic aerogels as sorbent materials for the removal of organic dyes from aqueous solutions." Journal of the American Ceramic Society 101, no. 2 (2018): 821-830.
|
-
Research Citations of ACS Material Products
- Luo, Sheng, et al. “Confinement-Induced Supercriticality and Phase Equilibria of Hydrocarbons in Nanopores.” Langmuir, vol. 32, no. 44, 2016, pp. 11506–11513., doi:10.1021/acs.langmuir.6b03177.
|
-
FAQ
1. What is the tap density and bulk density of the SiC Nanowire?
The tap density of our SiC Nanowire is 3.2g/cm³ and the bulk density is 0.1g/cm³
|
-
FAQ
1. What dispersants can be used to disperse Silicon Nanoparticles? Silicon nanoparticles can be dipersed in water or ethanol.
2. Which solvent can be used to dissolve Silicon Nanoparticles? You will need to ultrasonicate it with 40% hydrofluoric acid.
|
-
Research Citations of ACS Material Products
- Luo, Wen, and Spyridon Zafeiratos. “Graphene-Coated ZnO and SiO2 as Supports for CoO Nanoparticles with Enhanced Reducibility (ChemPhysChem 19/2016).” ChemPhysChem, vol. 17, no. 19, Apr. 2016, pp. 3146–3146., doi:10.1002/cphc.201601023.
- Nieto, Andy, et al. “Graphene reinforced metal and ceramic matrix composites: a review.” International Materials Reviews, vol. 62, no. 5, 27 Oct. 2016, doi:10.1080/09506608.2016.1219481.
|
-
Research Citations of ACS Material Products
- Gerardo, Carlos, et al. “Fabrication of Circuits on Flexible Substrates Using Conductive SU-8 for Sensing Applications.” Sensors, vol. 17, no. 7, 2017, p. 1420., doi:10.3390/s17061420.
- Pouran, Hamid M. "Engineered Nanomaterials in the Environment, their Potential Fate and Behaviour and Emerging Techniques to Measure Them." Handbook of Environmental Materials Management (2018): 1-15.
|
-
FAQ
1. What is the stabilizing agent used for your Silver Nanowire and what is the wt% left in the final product?
We only use Polyvinylpyrrolidone (PVP) and there is <0.5wt% left in our final product.
2. What geometric shape is the cross-section of your Silver Nanowires?
The cross-section of our silver nanowire is pentagonal.
Research Citations of ACS Material Products
- Li, Xiaokai, et al. “Device Area Scale-Up and Improvement of SWNT/Si Solar Cells Using Silver Nanowires.” Advanced Energy Materials, vol. 4, no. 12, 26 Aug. 2014, doi:10.1002/aenm.201400186.
- Lowenthal, Mark David, et al. Metallic nanofiber ink, substantially transparent conductor, and fabrication method.
- Kandare, Everson, et al. “Improving the through-Thickness thermal and electrical conductivity of carbon fibre/Epoxy laminates by exploiting synergy between graphene and silver nano-Inclusions.” Composites Part A: Applied Science and Manufacturing, vol. 69, 2015, pp. 72–82., doi:10.1016/j.compositesa.2014.10.024.
- Al-Daffaie, Shihab, et al. “1-D and 2-D Nanocontacts for Reliable and Efficient Terahertz Photomixers.” IEEE Transactions on Terahertz Science and Technology, vol. 5, no. 3, 2015, pp. 398–405., doi:10.1109/tthz.2015.2399772.
- Bari, Bushra, et al. “MeV carbon ion irradiation-Induced changes in the electrical conductivity of silver nanowire networks.” Current Applied Physics, vol. 15, no. 5, 2015, pp. 642–647., doi:10.1016/j.cap.2015.02.023.
- Zhu, Zhuan, et al. “Generation and Detection of Surface Plasmon Polaritons by Transition Metal Dichalcogenides for Chip-Level Electronic-Photonic Integrated Circuits.” ACS Photonics, 7 July 2015, doi:10.1021/acsphotonics.6b00101.
- Shehla, Honey, et al. “Protons Irradiation Induced Coalescence of Silver Nanowires.” Current Nanoscience, vol. 11, no. 6, Oct. 2015, pp. 792–796., doi:10.2174/1573413711666150430223538.
- Shehla, H., et al. “Fabrication of Amorphous Silver Nanowires by Helium Ion Beam Irradiation.” Chinese Physics Letters, vol. 32, no. 9, 2015, p. 096101., doi:10.1088/0256-307x/32/9/096101.
- Cheng, Zhe, et al. “Strongly Anisotropic Thermal and Electrical Conductivities of Self-Assembled Silver Nanowire Network.” RSC Advances, pp. 90674–90681., doi:10.1039/C6RA20331K.
- S, Honey, et al. “Large scale silver nanowires network fabricated by MeV hydrogen (H ) ion beam irradiation.” Chinese Physics B, vol. 25, no. 4, 2016, p. 046105., doi:10.1088/1674-1056/25/4/046105.
- Zhu, Zhuan, et al. “Excitonic Resonant Emission–Absorption of Surface Plasmons in Transition Metal Dichalcogenides for Chip-Level Electronic–Photonic Integrated Circuits.” ACS Photonics, vol. 3, no. 5, 2016, pp. 869–874., doi:10.1021/acsphotonics.6b00101.
- Shehla, Honey, et al. “Silver Nanowires Stability and Burying into Substrates Under MeV Proton Irradiation.” Current Nanoscience, vol. 12, no. 6, 2016, pp. 774–780., doi:10.2174/1573413712666160616090649.
- Jeong, Soon Moon, et al. “Stretchable, alternating-Current-Driven white electroluminescent device based on bilayer-Structured quantum-Dot-Embedded polydimethylsiloxane elastomer.” RSC Advances, vol. 7, no. 15, 2017, pp. 8816–8822., doi:10.1039/c7ra00195a.
- Alami, Abdul Hai, et al. “Assessment of silver nanowires infused with zinc oxide as a transparent electrode for dye-Sensitized solar cell applications.” Energy, vol. 139, 2017, pp. 1231–1236., doi:10.1016/j.energy.2017.03.171.
- Pinto, Thassyo, et al. “CNT-Based sensor arrays for local strain measurements in soft pneumatic actuators.” International Journal of Intelligent Robotics and Applications, vol. 1, no. 2, 2017, pp. 157–166., doi:10.1007/s41315-017-0018-6.
- Nguyen, Quoc K. “A flexible capacitance sensor integrated micropump for precise volume control.” Thesis / Dissertation ETD, May 2017.
- Cheng, Fei, et al. “Enhanced Photoluminescence of Monolayer WS2 on Ag Films and Nanowire–WS2–Film Composites.” ACS Photonics, vol. 4, no. 6, 2017, pp. 1421–1430., doi:10.1021/acsphotonics.7b00152.
- Yao, Shanshan, et al. “Soft electrothermal actuators using silver nanowire heaters.” Nanoscale, vol. 9, no. 11, 2017, pp. 3797–3805., doi:10.1039/c6nr09270e.
- Altinkok, Cagatay, et al. “Cysteamine-Functionalized silver nanowires as hydrogen donor for type II photopolymerization.” Journal of Photochemistry and Photobiology A: Chemistry, vol. 346, 2017, pp. 479–484., doi:10.1016/j.jphotochem.2017.06.035.
- Albano, Luiz G.s., et al. “Low voltage and high frequency vertical organic field effect transistor based on rod-Coating silver nanowires grid electrode.” Organic Electronics, vol. 50, 2017, pp. 311–316., doi:10.1016/j.orgel.2017.08.011.
- Kim, Hae-Jin, et al. “Rubbery electronics and sensors from intrinsically stretchable elastomeric composites of semiconductors and conductors.” Science Advances, vol. 3, no. 9, 2017, doi:10.1126/sciadv.1701114.
- Cai, Le, et al. “Direct Printing for Additive Patterning of Silver Nanowires for Stretchable Sensor and Display Applications.” Advanced Materials Technologies, 2017, p. 1700232., doi:10.1002/admt.201700232.
- Kim, Hae-Jin, et al. “Highly Sensitive and Very Stretchable Strain Sensor Based on a Rubbery Semiconductor.” ACS Applied Materials & Interfaces, 2018, doi:10.1021/acsami.7b17709.
- Bellet, Daniel, et al. “Transparent Electrodes Based on Silver Nanowire Networks: From Physical Considerations towards Device Integration.” Materials, vol. 10, no. 6, 2017, p. 570., doi:10.3390/ma10060570.
- Teymouri, Arastoo, et al. “Low-Temperature Solution Processed Random Silver Nanowire as a Promising Replacement for Indium Tin Oxide.” ACS Applied Materials & Interfaces, vol. 9, no. 39, 2017, pp. 34093–34100., doi:10.1021/acsami.7b13085.
- Dexter, M., et al. “Controlling processing temperatures and self-Limiting behaviour in intense pulsed sintering by tailoring nanomaterial shape distribution.” RSC Advances, vol. 7, no. 89, 2017, pp. 56395–56405., doi:10.1039/c7ra11013h.
- Kumar, D, et al. “Mixed-Dimension silver nanowires for solution-Processed, flexible, transparent and conducting electrodes with improved optical and physical properties.” Flexible and Printed Electronics, vol. 2, no. 1, Jan. 2017, p. 015005., doi:10.1088/2058-8585/aa6011.
- Camic, B. Tugba, et al. “Fabrication of a transparent conducting electrode based on graphene/Silver nanowires via layer-by-Layer method for organic photovoltaic devices.” Journal of Colloid and Interface Science, vol. 505, 2017, pp. 79–86., doi:10.1016/j.jcis.2017.05.065.
- Ding, Z., et al. “Spray coated silver nanowires as transparent electrodes in OPVs for Building Integrated Photovoltaics applications.” Solar Energy Materials and Solar Cells, vol. 157, 2016, pp. 305–311., doi:10.1016/j.solmat.2016.05.053.
- Camic, B. Tugba, et al. “Solution-Processable transparent conducting electrodes via the self-Assembly of silver nanowires for organic photovoltaic devices.” Journal of Colloid and Interface Science, vol. 512, 2018, pp. 158–164., doi:10.1016/j.jcis.2017.09.112.
- Oytun, Faruk, et al. “Fabrication of solution-Processable, highly transparent and conductive electrodes via layer-by-Layer assembly of functional silver nanowires.” Thin Solid Films, vol. 636, 2017, pp. 40–47., doi:10.1016/j.tsf.2017.05.029.
- Deignan, Geoffrey, and Irene A. Goldthorpe. “The dependence of silver nanowire stability on network composition and processing parameters.” RSC Advances, vol. 7, no. 57, 2017, pp. 35590–35597., doi:10.1039/c7ra06524h.
- Alami, Abdul Hai, et al. “Assessment of silver nanowires infused with zinc oxide as a transparent electrode for dye-Sensitized solar cell applications.” Energy, vol. 139, 2017, pp. 1231–1236., doi:10.1016/j.energy.2017.03.171.
- Lee, Kyung Min. “Enhanced outcoupling in flexible organic light-Emitting diodes on scattering polyimide substrates.” Organic Electronics, vol. 51, Dec. 2017, pp. 471–476., doi:10.1016/j.orgel.2017.09.042.
- Ishaq, Ahmad, et al. “Improvement of optical transmittance and electrical conductivity of silver nanowires by Cu ion beam irradiation.” Materials Research Express, vol. 4, no. 7, 2017, p. 075055., doi:10.1088/2053-1591/aa7e60.
- Pantoja, Elisa, et al. “Low thermal emissivity surfaces using AgNW thin films.” Nanotechnology, vol. 28, no. 50, 2017, p. 505708., doi:10.1088/1361-6528/aa96c2.
- Ricciardulli, Antonio Gaetano, Sheng Yang, Gert‐Jan AH Wetzelaer, Xinliang Feng, and Paul WM Blom. "Hybrid Silver Nanowire and Graphene‐Based Solution‐Processed Transparent Electrode for Organic Optoelectronics." Advanced Functional Materials 28, no. 14 (2018): 1706010.
- Guo, Chuanrui, Liang Fan, Chenglin Wu, Genda Chen, and Wei Li. "Ultrasensitive LPFG corrosion sensor with Fe-C coating electroplated on a Gr/AgNW film." Sensors and Actuators B: Chemical 283 (2019): 334-342.
- Li, Yanxiao, Congjie Wei, and Chenglin Wu. "Adhesion of silver nano wire graphene composite film." Journal of colloid and interface science 535 (2019): 341-352.
- Shin, Dong Hee, Gyea Young Kwak, Jong Min Kim, Chan Wook Jang, Suk-Ho Choi, and Kyung Joong Kim. "Remarkable enhancement of stability in high-efficiency Si-quantum-dot heterojunction solar cells by employing bis (trifluoromethanesulfonyl)-amide as a dopant for graphene transparent conductive electrodes." Journal of Alloys and Compounds 773 (2019): 913-918.
- Shin, Dong Hee, Chan Wook Jang, Jong Min Kim, and Suk-Ho Choi. "Self-powered Ag-nanowires-doped graphene/Si quantum dots/Si heterojunction photodetectors." Journal of Alloys and Compounds 758 (2018): 32-37.
- Albano, Luiz Gustavo Simão, João Vitor Paulin, Luciana Daniele Trino, Silvia Leticia Fernandes, and Carlos Frederico de Oliveira Graeff. "Ultraviolet‐protective thin film based on PVA–melanin/rod‐coated silver nanowires and its application as a transparent capacitor." Journal of Applied Polymer Science (2019): 47805.
- Shi, Ge, Tianqing Liu, Zlatko Kopecki, Allison Cowin, Ivan Lee, Jing-Hong Pai, Sean E. Lowe, and Yu Lin Zhong. "A Multifunctional Wearable Device with a Graphene/Silver Nanowire Nanocomposite for Highly Sensitive Strain Sensing and Drug Delivery." C 5, no. 2 (2019): 17.
- Bao, Jiming, Zhuan Zhu, and Zhiming Wang. "A method to fabricate chip-scale electronic photonic (plasmonic)-integrated circuits." U.S. Patent Application 15/736,941, filed June 21, 2018.
- Chen, Yiting. "Development and Integration of Stretchable Electronic Components into Light-Emitting Devices. pdf." (2018).
- Albano, Luíz Gustavo Simão. "Estudo de materiais e dispositivos para eletrônica orgânica." (2018).
- Lee, Joong Hoon, Ji-Young Hwang, Jia Zhu, Ha Ryeon Hwang, Seung Min Lee, Huanyu Cheng, Sang-Hoon Lee, and Suk-Won Hwang. "Flexible conductive composite integrated with personal earphone for wireless, real-time monitoring of electrophysiological signs." ACS applied materials & interfaces 10, no. 25 (2018): 21184-21190.
- Sim, Kyoseung, Zhoulyu Rao, Hae-Jin Kim, Anish Thukral, Hyunseok Shim, and Cunjiang Yu. "Fully rubbery integrated electronics from high effective mobility intrinsically stretchable semiconductors." Science advances 5, no. 2 (2019): eaav5749.
- Zharfan, Muhammad, and Mohd Halizan. "Integration of nanoporous structure into vertical organic field effect transistor." PhD diss., University of Malaya, 2018.
- Song, Seongkyu, Hyunseok Shim, Sang Kyoo Lim, and Soon Moon Jeong. "Patternable and Widely Colour-Tunable Elastomer-Based Electroluminescent Devices." Scientific reports 8, no. 1 (2018): 3331.
- Lee, Sangyeob, Jun Su Lee, Jiseong Jang, Ki-Ha Hong, Doh-Kwon Lee, Soomin Song, Kihwan Kim et al. "Robust nanoscale contact of silver nanowire electrodes to semiconductors to achieve high performance chalcogenide thin film solar cells." Nano energy 53 (2018): 675-682.
- Kwak, Dong Yeung, Ramon C. Cancel Olmo, and L. I. Jue. "Silver nanowire touch sensor component." U.S. Patent Application 14/915,148, filed February 1, 2018.
- Chellattoan, Ragesh, Vinicius Lube, and Gilles Lubineau. "Toward Programmable Materials for Wearable Electronics: Electrical Welding Turns Sensors into Conductors." Advanced Electronic Materials 5, no. 1 (2019): 1800273.
- Bellan, Leon, and Xin Zhang. "Transient electronics using thermoresponsive materials." U.S. Patent Application 15/817,873, filed June 21, 2018.
- Shi, Ge, Tianqing Liu, Zlatko Kopecki, Allison Cowin, Ivan Lee, Jing-Hong Pai, Sean E. Lowe, and Yu Lin Zhong. "A Multifunctional Wearable Device with a Graphene/Silver Nanowire Nanocomposite for Highly Sensitive Strain Sensing and Drug Delivery." C 5, no. 2 (2019): 17.
|
-
FAQ
1. How to disperse the Single Layer Graphene Powder into Aqueous Systems?
The SLG product produced by ACS Material has no functional groups on the surface‚ and it can be dispersed in water or ethanol with a dispersion aid. These two dispersion aids have been proven to be useful:
1) Sodium dodecylbenzene sulfonate (SDBS) 2) Sodium dodecyl sulfate (SDS)
Add dispersant to water‚ and then add single layer graphene with the use of an ultrasonic mixer. The addition rate of single layer graphene should be carefully controlled until the desired concentration is achieved. Experiments are needed to determine the appropriate quantity of dispersant in your system‚ but in our experience with water‚ 10mg/ml dispersant is preferred. The sonication time should be 15+ minutes. If you notice some settling‚ assuming you have not attempted to over-concentrate the dispersion‚ the graphene can be redispersed with the aid of sonication. The maximum concentration of single layer graphene in water is about 0.1 mg/ml with SDBS as dispersant‚ or 100mg/l.
2. Are there other elements in Single Layer Graphene?
Single Layer Graphene contains a bit of sulfur. Sulfuric acid is necessary for intercalation during the initial treatment and sulfur cannot be completely removed in the final product.
Research Citations of ACS Material Products
- Randeniya, Lakshman K., et al. “Harnessing the Influence of Reactive Edges and Defects of Graphene Substrates for Achieving Complete Cycle of Room-Temperature Molecular Sensing.” Small, vol. 9, no. 23, Jan. 2013, pp. 3993–3999., doi:10.1002/smll.201300689.
- Zhang, Lv, et al. “A tough graphene nanosheet/Hydroxyapatite composite with improved in vitro biocompatibility.” Carbon, vol. 61, 2013, pp. 105–115., doi:10.1016/j.carbon.2013.04.074.
- Li, Yunyong, et al. “Simultaneous Formation of Ultrahigh Surface Area and Three-Dimensional Hierarchical Porous Graphene-Like Networks for Fast and Highly Stable Supercapacitors.” Advanced Materials, vol. 25, no. 17, 2013, pp. 2474–2480., doi:10.1002/adma.201205332.
- Xu, Peng, et al. “Synergy among binary (MWNT, SLG) nano-Carbons in polymer nano-Composites: a Raman study.” Nanotechnology, vol. 23, no. 31, 2012, p. 315706., doi:10.1088/0957-4484/23/31/315706.
- Loomis, James, and Balaji Panchapakesan. “Dimensional dependence of photomechanical response in carbon nanostructure composites: a case for carbon-Based mixed-Dimensional systems.” Nanotechnology, vol. 23, no. 21, Mar. 2012, p. 215501., doi:10.1088/0957-4484/23/21/215501.
- Ip, Alexander C.-F., et al. “Oxidation Level-Dependent Zwitterionic Liposome Adsorption and Rupture by Graphene-Based Materials and Light-Induced Content Release.” Small, vol. 9, no. 7, 2012, pp. 1030–1035., doi:10.1002/smll.201202710.
- Xu, Peng, et al. “Load transfer and mechanical properties of chemically reduced graphene reinforcements in polymer composites.” Nanotechnology, vol. 23, no. 50, 2012, p. 505713., doi:10.1088/0957-4484/23/50/505713.
- Loomis, James, et al. “Layer dependent mechanical responses of graphene composites to near-Infrared light.” Applied Physics Letters, vol. 100, no. 7, 2012, p. 073108., doi:10.1063/1.3685479.
- Loomis, James, and Balaji Panchapakesan. “Large photocurrents in single layer graphene thin films: effects of diffusion and drift.” Nanotechnology, vol. 23, no. 26, 2012, p. 265203., doi:10.1088/0957-4484/23/26/265203.
- Chen, Qi, et al. “Enhanced Hot-Carrier Luminescence in Multilayer Reduced Graphene Oxide Nanospheres.” Scientific Reports, vol. 3, no. 1, 2013, doi:10.1038/srep02315.
- Xu, Peng, et al. “Synergy among binary (MWNT, SLG) nano-Carbons in polymer nano-Composites: a Raman study.” Nanotechnology, vol. 23, no. 31, 2012, p. 315706., doi:10.1088/0957-4484/23/31/315706.
- Aziz, Saad, et al. “Nanoarchitectured LiMn2O4/Graphene/ZnO Composites as Electrodes for Lithium Ion Batteries.” Journal of Materials Science & Technology, vol. 30, no. 5, 2014, pp. 427–433., doi:10.1016/j.jmst.2014.03.007.
- Lewandowski, Andrzej, et al. “Capacity of graphene anode in ionic liquid electrolyte.” Journal of Solid State Electrochemistry, vol. 18, no. 10, 2014, pp. 2781–2788., doi:10.1007/s10008-014-2539-3.
- Zuccon, Sara, et al. “Functional palladium metal films for plasmonic devices: an experimental proof.” Journal of Optics, vol. 16, no. 5, 2014, p. 055001., doi:10.1088/2040-8978/16/5/055001.
- Tampieri, Francesco, et al. “A comparative electron paramagnetic resonance study of expanded graphites and graphene.” J. Mater. Chem. C, vol. 2, no. 38, 2014, pp. 8105–8112., doi:10.1039/c4tc01383b.
- Sharma, Rajni, et al. “ZnO anchored graphene hydrophobic nanocomposite-Based bulk heterojunction solar cells showing enhanced short-circuit current.” Journal of Materials Chemistry C, 1 Aug. 2014, pp. 8142–8151., doi:10.1039/C4TC01056F.
- Majidian, Maryam, et al. “Electrical conduction of photo-Patternable SU8–graphene composites.” Carbon, vol. 80, 2014, pp. 364–372., doi:10.1016/j.carbon.2014.08.075.
- Zhang, Tao, et al. “Gel-Derived Cation-π Stacking Films of Carbon Nanotube-Graphene Complexes as Oxygen Cathodes.” ChemSusChem, vol. 7, no. 10, 2014, pp. 2845–2852., doi:10.1002/cssc.201402567.
- Swiderska-Mocek, Agnieszka, and Ewelina Rudnicka. “Lithium–sulphur battery with activated carbon cloth-Sulphur cathode and ionic liquid as electrolyte.” Journal of Power Sources, vol. 273, 2015, pp. 162–167., doi:10.1016/j.jpowsour.2014.09.020.
- Terborg, Lydia, et al. “Porous polymer monoliths with incorporated single layer graphene.” Scientia Chromatographica, vol. 6, no. 1, 2014, pp. 27–33., doi:10.4322/sc.2014.017.
- Sims, Christopher M., et al. “CO tolerance of Pt and PtSn intermetallic electrocatalysts on synthetically modified reduced graphene oxide supports.” Dalton Transactions, vol. 44, no. 3, 2015, pp. 977–987., doi:10.1039/c4dt02544j.
- Amoli, Behnam Meschi, et al. “Highly electrically conductive adhesives using silver nanoparticle (Ag NP)-Decorated graphene: the effect of NPs sintering on the electrical conductivity improvement.” Journal of Materials Science: Materials in Electronics, vol. 26, no. 1, Apr. 2014, pp. 590–600., doi:10.1007/s10854-014-2440-y.
- Amoli, Behnam Meschi, et al. “SDS-Stabilized graphene nanosheets for highly electrically conductive adhesives.” Carbon, vol. 91, 2015, pp. 188–199., doi:10.1016/j.carbon.2015.04.039.
- Wehnert, F., et al. “Design of multifunctional adhesives by the use of carbon nanoparticles.” Journal of Adhesion Science and Technology, vol. 29, no. 17, 2015, pp. 1849–1859., doi:10.1080/01694243.2015.1014536.
- Liang, Xiao, et al. “A highly efficient polysulfide mediator for lithium–sulfur batteries.” Nature Communications, vol. 6, June 2015, p. 5682., doi:10.1038/ncomms6682.
- Paczosa-Bator, Beata. “Ion-Selective electrodes with superhydrophobic polymer/Carbon nanocomposites as solid contact.” Carbon, vol. 95, 2015, pp. 879–887., doi:10.1016/j.carbon.2015.09.006.
- Bhatnagar, Deepika, et al. “Graphene quantum dots FRET based sensor for early detection of heart attack in human.” Biosensors and Bioelectronics, vol. 79, 2016, pp. 495–499., doi:10.1016/j.bios.2015.12.083.
- Saha, Dipendu, et al. “Adsorption of CO2, CH4, and N2 in Micro-Mesoporous Nanographene: A Comparative Study.” Journal of Chemical & Engineering Data, vol. 60, no. 9, Nov. 2015, pp. 2636–2645., doi:10.1021/acs.jced.5b00291.
- Saha, Dipendu, et al. “A study on the cytotoxicity of carbon-based materials.” Materials Science and Engineering: C , vol. 68, Nov. 2016, pp. 101–108., doi:10.1016/j.msec.2016.05.094.
- Alhumade, H., et al. “Enhanced protective properties and UV stability of epoxy/Graphene nanocomposite coating on stainless steel.” Express Polymer Letters, vol. 10, no. 12, 2016, pp. 1034–1046., doi:10.3144/expresspolymlett.2016.96.
- Deng, Wenfang, et al. “Three-Dimensional graphene-like carbon frameworks as a new electrode material for electrochemical determination of small biomolecules.” Biosensors and Bioelectronics, vol. 85, 2016, pp. 618–624., doi:10.1016/j.bios.2016.05.065.
- Alhumade, Hesham, et al. “Corrosion inhibition of copper in sodium chloride solution using polyetherimide/Graphene composites.” The Canadian Journal of Chemical Engineering, vol. 94, no. 5, 2016, pp. 896–904., doi:10.1002/cjce.22439.
- Pięk, Magdalena, et al. “The Complex Crystal of NaTCNQ–TCNQ Supported on Different Carbon Materials as Ion-to-Electron Transducer in All-Solid-State Sodium-Selective Electrode.” Journal of The Electrochemical Society, vol. 163, no. 13, 2016, doi:10.1149/2.0341613jes.
- Albelda, Jasmine A.V, et al. “Graphene-Titanium dioxide nanocomposite based hypoxanthine sensor for assessment of meat freshness.” Biosensors and Bioelectronics, vol. 89, 2017, pp. 518–524., doi:10.1016/j.bios.2016.03.041.
- Dubey, Nileshkumar, et al. “Graphene: An Emerging Carbon Nanomaterial for Bone Tissue Engineering.” Graphene-Based Materials in Health and Environment Carbon Nanostructures, 2016, pp. 135–158., doi:10.1007/978-3-319-45639-3_5.
- Dobrota, Ana S., et al. “Stabilization of alkali metal ions interaction with OH-Functionalized graphene via clustering of OH groups – implications in charge storage applications.” RSC Advances, vol. 6, no. 63, 2016, pp. 57910–57919., doi:10.1039/c6ra13509a.
- Mohan, Balaji, et al. “Mechanochemical Synthesis of Active Magnetite Nanoparticles Supported on Charcoal for Facile Synthesis of Alkynyl Selenides by C−H Activation.” ChemCatChem, vol. 8, no. 14, 2016, pp. 2345–2350., doi:10.1002/cctc.201600280.
- Pięk, Magdalena, et al. “All-Solid-State nitrate selective electrode with graphene/Tetrathiafulvalene nanocomposite as high redox and double layer capacitance solid contact.” Electrochimica Acta, vol. 210, 2016, pp. 407–414., doi:10.1016/j.electacta.2016.05.170.
- Marinoiu, A., et al. “Iodinated carbon materials for oxygen reduction reaction in proton exchange membrane fuel cell. Scalable synthesis and electrochemical performances.” Arabian Journal of Chemistry, 2016, doi:10.1016/j.arabjc.2016.12.002.
- Pu, Zhihua, et al. “A continuous glucose monitoring device by graphene modified electrochemical sensor in microfluidic system.” Biomicrofluidics, vol. 10, no. 1, 2016, p. 011910., doi:10.1063/1.4942437.
- Gatti, Teresa, et al. “Boosting Perovskite Solar Cells Performance and Stability through Doping a Poly-3(Hexylthiophene) Hole Transporting Material with Organic Functionalized Carbon Nanostructures.” Advanced Functional Materials, vol. 26, no. 41, 2016, pp. 7443–7453., doi:10.1002/adfm.201602803.
- Nieto, Andy, et al. “Graphene reinforced metal and ceramic matrix composites: a review.” International Materials Reviews, vol. 62, no. 5, 2016, pp. 241–302., doi:10.1080/09506608.2016.1219481.
- Marinoiu, Adriana, et al. “Doped Graphene as Non-Metallic Catalyst for Fuel Cells.” Materials Science, vol. 23, no. 2, 2017, doi:10.5755/j01.ms.23.2.16216.
- Kang, Shin Wook, et al. “High-Performance Fe 5 C 2 @CMK-3 nanocatalyst for selective and high-Yield production of gasoline-Range hydrocarbons.” Journal of Catalysis, vol. 349, 2017, pp. 66–74., doi:10.1016/j.jcat.2017.03.004.
- Pięk, Magdalena, et al. “High selective potentiometric sensor for determination of nanomolar con-Centration of Cu(II) using a polymeric electrode modified by a graphene/7,7,8,8-Tetracyanoquinodimethane nanoparticles.” Talanta, vol. 170, 2017, pp. 41–48., doi:10.1016/j.talanta.2017.03.068.
- Marinoiu, Adriana, et al. “Low cost iodine intercalated graphene for fuel cells electrodes.” Applied Surface Science, vol. 424, 2017, pp. 93–100., doi:10.1016/j.apsusc.2017.01.295.
- Liu, Yangshuai, et al. “Asymmetric supercapacitor, based on composite MnO 2 -Graphene and N-Doped activated carbon coated carbon nanotube electrodes.” Electrochimica Acta, vol. 233, 2017, pp. 142–150., doi:10.1016/j.electacta.2017.03.028.
- Huang, Kun, et al. “Graphene coupled with Pt cubic nanoparticles for high performance, air-Stable graphene-Silicon solar cells.” Nano Energy, vol. 32, 2017, pp. 225–231., doi:10.1016/j.nanoen.2016.12.042.
- Deng, Wenfang, et al. “Three-Dimensional nitrogen-Doped graphene derived from poly-o-Phenylenediamine for high-Performance supercapacitors.” Journal of Electroanalytical Chemistry, vol. 787, 2017, pp. 103–109., doi:10.1016/j.jelechem.2017.01.047.
- Müller, Michael, et al. “Effect of Graphite Nanoplate Morphology on the Dispersion and Physical Properties of Polycarbonate Based Composites.” Materials, vol. 10, no. 6, 2017, p. 545., doi:10.3390/ma10050545.
- Chakrabarti, Barun, et al. “Enhanced Performance of an All-Vanadium Redox Flow Battery Employing Graphene Modified Carbon Paper Electrodes.” International Journal of Chemical and Molecular Engineering, vol. 11, no. 9, 2017, doi:10.1999/1307-6892/10007796.
- Gatti, Teresa, et al. “A D-π-A organic dye – Reduced graphene oxide covalent dyad as a new concept photosensitizer for light harvesting applications.” Carbon, vol. 115, 2017, pp. 746–753., doi:10.1016/j.carbon.2017.01.081.
- Zhou, Yikang, et al. “Graphene-Doped polyaniline nanocomposites as electromagnetic wave absorbing materials.” Journal of Materials Science: Materials in Electronics, vol. 28, no. 15, May 2017, pp. 10921–10928., doi:10.1007/s10854-017-6872-z.
- Das, Suprem R., et al. “Electrical Differentiation of Mesenchymal Stem Cells into Schwann-Cell-Like Phenotypes Using Inkjet-Printed Graphene Circuits.” Advanced Healthcare Materials, vol. 6, no. 7, 20 Feb. 2017, doi:10.1002/adhm.201601087.
- Kafiah, Feras, et al. “Synthesis of Graphene Based Membranes: Effect of Substrate Surface Properties on Monolayer Graphene Transfer.” Materials, vol. 10, no. 1, 2017, p. 86., doi:10.3390/ma10010086.
- Marinoiu, Adriana, et al. “Doped Graphene as Non-Metallic Catalyst for Fuel Cells.” Materials Science, vol. 23, no. 2, 2017, doi:10.5755/j01.ms.23.2.16216.
- Casalino, M., et al. “Free-Space graphene/Silicon photodetectors operating at 2 micron.” 15 Nov. 2017.
- Pu, Zhihua, et al. “A Wearable Continuous Glucose Monitoring System with Electrochemical Sensor Modified by Graphene.” Oct. 2015.
- Alhumade, Hesham, et al. “Proceedings of the 2016 International Conference on Industrial Engineering and Operations Management.” Optimizing Corrosion Protection of Stainless Steel 304 by Epoxy-Graphene Composite using Factorial Experimental Design.
- Slamet, and Raudina. “Degradation of 2,4,6-Trichlorophenol and hydrogen production simultaneously by TiO2 nanotubes/Graphene composite.” 2017, doi:10.1063/1.5011931.
- Marinoiu, Adriana, et al. “Low cost iodine intercalated graphene for fuel cells electrodes.” Applied Surface Science, North-Holland, 31 Jan. 2017, www.sciencedirect.com/science/article/pii/S0169433217303227.
- Ha, Trung B., et al. “Electro-Immobilization of Acetylcholinesterase Using Polydopamine for Carbaryl Microsensor.” Journal of Electronic Materials, vol. 47, no. 2, 2017, pp. 1686–1693., doi:10.1007/s11664-017-5880-3.
- Guarracino, Paola, et al. “Probing photoinduced electron-Transfer in graphene–dye hybrid materials for DSSC.” Phys. Chem. Chem. Phys., vol. 19, no. 40, 2017, pp. 27716–27724., doi:10.1039/c7cp04308b.
- Lu, Bingyu, et al. “Side-Polished fiber SPR sensor with tempetrature self-Compensation for continuous glucose monitoring.” 2016 IEEE 29th International Conference on Micro Electro Mechanical Systems (MEMS), 2016, doi:10.1109/memsys.2016.7421648.
- Huang, Kun, et al. “High and Fast Response of a Graphene-Silicon Photodetector Coupled with 2D Fractal Platinum Nanoparticles.” Advanced Optical Materials, vol. 6, no. 1, Jan. 2017, p. 1700793., doi:10.1002/adom.201700793.
- Hondred, John A., et al. “High-Resolution Graphene Films for Electrochemical Sensing via Inkjet Maskless Lithography.” ACS Nano, vol. 11, no. 10, 2017, pp. 9836–9845., doi:10.1021/acsnano.7b03554.
- Seehra, Mohindar S., et al. “Correlation between X-Ray diffraction and Raman spectra of 16 commercial graphene–based materials and their resulting classification.” Carbon, vol. 111, 2017, pp. 380–385., doi:10.1016/j.carbon.2016.10.010.
- Yadav, Shriniwas, and Inderpreet Kaur. “Low temperature processed graphene thin film transparent electrodes for supercapacitor applications.” RSC Advances, vol. 6, no. 82, 2016, pp. 78702–78713., doi:10.1039/c6ra17668b.
- He, Qing, et al. “Enabling Inkjet Printed Graphene for Ion Selective Electrodes with Postprint Thermal Annealing.” ACS Applied Materials & Interfaces, vol. 9, no. 14, 2017, pp. 12719–12727., doi:10.1021/acsami.7b00092.
- Barbon, Antonio, and Francesco Tampieri. “Identification of slow relaxing spin components by pulse EPR techniques in graphene-Related materials.” AIMS Materials Science, vol. 4, no. 1, 2017, pp. 147–157., doi:10.3934/matersci.2017.1.147.
- Ali, Nafisa, Priyabrata Pal, Fawzi Banat, and Chandrasekar Srinivasakannan. "Selective removal of diethanolamine from methyldiethanolamine solution using chemically reduced single-layer graphene and activated carbon." Separation Science and Technology (2018): 1-11.
- Casalino, Maurizio, Roberto Russo, Carmela Russo, Anna Ciajolo, Emiliano Di Gennaro, Mario Iodice, and Giuseppe Coppola. "Free-Space Schottky Graphene/Silicon Photodetectors Operating at 2 μm." ACS Photonics 5, no. 11 (2018): 4577-4585.
- Paustovsky, A. V., V. E. Sheludko, E. Ya Telnikov, A. K. Marchuk, V. V. Kremenitsky, O. P. Tarasyuk, and S. P. Rogalsky. "The Structure and Electrical Properties of Graphene-Containing Thick Films." Powder Metallurgy and Metal Ceramics 57, no. 5-6 (2018): 285-292.
- Hoeprich Jr, Paul D., and Sangil Kim. "Electrochemical flow-cell for hydrogen production and nicotinamide dependent target reduction, and related methods and systems." U.S. Patent Application 14/861,750, filed March 24, 2016.
- Kinastowska, K., K. Piela, M. Gordel, A. Żak, R. Kołkowski, and M. Samoć. "Gold nanoparticle-decorated graphene as a nonlinear optical material in the visible and near-infrared spectral range." Physical Chemistry Chemical Physics 20, no. 27 (2018): 18862-18872.
|
-
Research Citations of ACS Material Products
- Chaiyo, Sudkate, et al. “Electrochemical sensors for the simultaneous determination of zinc, cadmium and lead using a Nafion/Ionic liquid/Graphene composite modified screen-Printed carbon electrode.” Analytica Chimica Acta, vol. 918, 2016, pp. 26–34., doi:10.1016/j.aca.2016.03.026.
- Illakkiya, J. Tamil, et al. “Nebulized spray pyrolysis: a new method for synthesis of graphene film and their characteristics.” Surface and Coatings Technology, vol. 307, 2016, pp. 65–72., doi:10.1016/j.surfcoat.2016.08.051.
- Illakkiya, J. Tamil, et al. “Nanoarchitectured Semiconducting Photoelectrodes for Enhanced Stability and Photon Conversion Efficiency.” Carbon, vol. 111, 2017, pp. 713–721., doi:10.1016/j.carbon.2016.09.042.
- Nieto, Andy, et al. “Graphene reinforced metal and ceramic matrix composites: a review.” International Materials Reviews, vol. 62, no. 5, 2016, pp. 241–302., doi:10.1080/09506608.2016.1219481.
- Tiwari, Santosh Kr., et al. “Conductive Polymer Composites Based on Carbon Nanomaterials.” Springer Series on Polymer and Composite Materials Conducting Polymer Hybrids, Mar. 2016, pp. 117–142., doi:10.1007/978-3-319-46458-9_4.
- Chaiyo, Sudkate, et al. “Non-Enzymatic electrochemical detection of glucose with a disposable paper-Based sensor using a cobalt phthalocyanine–ionic liquid–graphene composite.” Biosensors and Bioelectronics, vol. 102, 2018, pp. 113–120., doi:10.1016/j.bios.2017.11.015.
- Lazarević-Pašti, Tamara, Vladan Anićijević, Miloš Baljozović, Dragana Vasić Anićijević, Sanjin Gutić, Vesna Vasić, Natalia V. Skorodumova, and Igor A. Pašti. "The impact of the structure of graphene-based materials on the removal of organophosphorus pesticides from water." Environmental Science: Nano (2018).
- Ali, Nafisa, Priyabrata Pal, Fawzi Banat, and Chandrasekar Srinivasakannan. "Selective removal of diethanolamine from methyldiethanolamine solution using chemically reduced single-layer graphene and activated carbon." Separation Science and Technology (2018): 1-11.
|
-
FAQ
1. What is the pH of Graphene Oxide Dispersion in Water?
The pH is 3 - 5.
2. What is the preparation process of Single Layer Graphene Oxide Dispersion in Water?
It is synthesized in water first and freeze dried to make powder, which is then dispersed in water at the required concentration.
3. Can ACS Material provide a higher concentration?
For a custom order, the maximum concentration we can offer for both water and ethanol dispersion is 10mg/ml. Customization fee and minimum order quantity may apply. Please contact us for more information.
4. What is the sonication period during the dispersion procedures?
The sonication period is about 30 minutes.
Research Citations of ACS Material Products
- Mukherjee, Rahul, et al. “Photothermally Reduced Graphene as High-Power Anodes for Lithium-Ion Batteries.” ACS Nano, vol. 6, no. 9, 2012, pp. 7867–7878., doi:10.1021/nn303145j.
- Chow, Philippe K., et al. “Mechanical Property Enhancement of Layered Reduced Graphene Oxide Papers by Non-Covalent Modification with Terephthalic Acid.” Particle & Particle Systems Characterization, vol. 31, no. 3, 2013, pp. 337–341., doi:10.1002/ppsc.201300206.
- Feng, Xuefei, et al. “Understanding the degradation mechanism of rechargeable lithium/Sulfur cells: a comprehensive study of the sulfur–graphene oxide cathode after discharge–charge cycling.” Phys. Chem., vol. 16, no. 32, 2014, pp. 16931–16940., doi:10.1039/c4cp01341g.
- Cui, Shumao, et al. “Ultrasensitive Chemical Sensing through Facile Tuning Defects and Functional Groups in Reduced Graphene Oxide.” Analytical Chemistry, vol. 86, no. 15, Nov. 2014, pp. 7516–7522., doi:10.1021/ac501274z.
- Mukherjee, Rahul, et al. “Defect-Induced plating of lithium metal within porous graphene networks.” Nature Communications, vol. 5, 2014, doi:10.1038/ncomms4710.
- Lammel, Tobias, et al. “Internalization and cytotoxicity of graphene oxide and carboxyl graphene nanoplatelets in the human hepatocellular carcinoma cell line Hep G2.” Particle and Fibre Toxicology, vol. 10, no. 1, 2013, p. 27., doi:10.1186/1743-8977-10-27.
- Cheng, Jianli, et al. “Self-Assembled V2O5 nanosheets/Reduced graphene oxide hierarchical nanocomposite as a high-Performance cathode material for lithium ion batteries.” Journal of Materials Chemistry A, vol. 1, no. 36, 2013, p. 10814., doi:10.1039/c3ta12066j.
- Li, Shanghao, et al. “Interaction between Graphene Oxide and Pluronic F127 at the Air–Water Interface.” Langmuir, vol. 29, no. 19, Feb. 2013, pp. 5742–5748., doi:10.1021/la401056t.
- Othman, Abdelhameed M., et al. “Enhancing selectivity in spectrofluorimetric determination of tryptophan by using graphene oxide nanosheets.” Analytica Chimica Acta, vol. 787, 2013, pp. 226–232., doi:10.1016/j.aca.2013.05.036.
- Zhang, Xu, et al. “Effects of Polyethylene Glycol on DNA Adsorption and Hybridization on Gold Nanoparticles and Graphene Oxide.” Langmuir, vol. 28, no. 40, 2012, pp. 14330–14337., doi:10.1021/la302799s.
- Li, Shanghao, et al. “Graphene Oxide as a Quencher for Fluorescent Assay of Amino Acids, Peptides, and Proteins.” ACS Applied Materials & Interfaces, vol. 4, no. 12, Mar. 2012, pp. 7069–7075., doi:10.1021/am302704a.
- Ip, Alexander C.-F., et al. “Oxidation Level-Dependent Zwitterionic Liposome Adsorption and Rupture by Graphene-Based Materials and Light-Induced Content Release.” Small, vol. 9, no. 7, 2012, pp. 1030–1035., doi:10.1002/smll.201202710.
- Chang, Jingbo, et al. “Ultrasonic-Assisted self-Assembly of monolayer graphene oxide for rapid detection of Escherichia coli bacteria.” Nanoscale, vol. 5, no. 9, 2013, p. 3620., doi:10.1039/c3nr00141e.
- Liu, Lin, et al. “Deposition and transport of graphene oxide in saturated and unsaturated porous media.” Chemical Engineering Journal, vol. 229, 1 Aug. 2013, pp. 444–449., doi:10.1016/j.cej.2013.06.030.
- Li, Shanghao, et al. “Head Groups of Lipids Govern the Interaction and Orientation between Graphene Oxide and Lipids.” The Journal of Physical Chemistry C, vol. 117, no. 31, 2013, pp. 16150–16158., doi:10.1021/jp405991q.
- Tran, H. V., et al. “Antibodies Directed to RNA/DNA Hybrids: An Electrochemical Immunosensor for MicroRNAs Detection using Graphene-Composite Electrodes.” Analytical Chemistry, vol. 85, no. 17, 2013, pp. 8469–8474., doi:10.1021/ac402154z.
- Liu, Biwu, et al. “Mechanisms of DNA Sensing on Graphene Oxide.” Analytical Chemistry, vol. 85, no. 16, June 2013, pp. 7987–7993., doi:10.1021/ac401845p.
- Luan, Xinning, et al. “Electrophoretic deposition of reduced graphene oxide nanosheets on TiO2 nanotube arrays for dye-Sensitized solar cells.” Electrochimica Acta, vol. 111, 2013, pp. 216–222., doi:10.1016/j.electacta.2013.08.016.
- Zhang, Ming, et al. “Synthesis of a multifunctional graphene–carbon nanotube aerogel and its strong adsorption of lead from aqueous solution.” RSC Advances, vol. 3, no. 43, 2013, p. 21099., doi:10.1039/c3ra44340j.
- Wu, Lei, et al. “Aggregation Kinetics of Graphene Oxides in Aqueous Solutions: Experiments, Mechanisms, and Modeling.” Langmuir, vol. 29, no. 49, Mar. 2013, pp. 15174–15181., doi:10.1021/la404134x.
- Wang, Feng, and Juewen Liu. “Nanodiamond decorated liposomes as highly biocompatible delivery vehicles and a comparison with carbon nanotubes and graphene oxide.” Nanoscale, vol. 5, no. 24, 2013, p. 12375., doi:10.1039/c3nr04143c.
- Zhang, Ming, et al. “Simple approach for large-Scale production of reduced graphene oxide films.” Chemical Engineering Journal, vol. 243, 2014, pp. 340–346., doi:10.1016/j.cej.2014.01.019.
- Frechette, M. F., et al. “Preparation and dielectric responses of solid epoxy composites containing a mixture of epoxy powder ball-Milled with GO.” 2013 Annual Report Conference on Electrical Insulation and Dielectric Phenomena, 2013, doi:10.1109/ceidp.2013.6748335.
- Chaturvedi, P. et al. “A nanoceria–platinum–graphene nanocomposite for electrochemical biosensing.” Biosensors and Bioelectronics, vol. 58, 15 Aug. 2014, doi:10.1016/j.bios.2014.02.021.
- Lammel, Tobias, and José M. Navas. “Graphene nanoplatelets spontaneously translocate into the cytosol and physically interact with cellular organelles in the fish cell line PLHC-1.” Aquatic Toxicology, vol. 150, 2014, pp. 55–65., doi:10.1016/j.aquatox.2014.02.016.
- Souza, Camille De, et al. “Electrocatalytic miRNA Detection Using Cobalt Porphyrin-Modified Reduced Graphene Oxide.” Sensors, vol. 14, no. 6, June 2014, pp. 9984–9994., doi:10.3390/s140609984.
- Lammel, Tobias, et al. “Potentiating effect of graphene nanomaterials on aromatic environmental pollutant-Induced cytochrome P450 1A expression in the topminnow fish hepatoma cell line PLHC-1.” Environmental Toxicology, vol. 30, no. 10, May 2014, pp. 1192–1204., doi:10.1002/tox.21991.
- Tran, H.V., et al. “An electrochemical ELISA-like immunosensor for miRNAs detection based on screen-Printed gold electrodes modified with reduced graphene oxide and carbon nanotubes.” Biosensors and Bioelectronics, vol. 62, 2014, pp. 25–30., doi:10.1016/j.bios.2014.06.014.
- Wang, Feng, and Juewen Liu. “Platinated DNA oligonucleotides: new probes forming ultrastable conjugates with graphene oxide.” Nanoscale, vol. 6, no. 12, 2014, p. 7079., doi:10.1039/c4nr00867g.
- Chen, Hao, et al. “Removal of sulfamethoxazole and ciprofloxacin from aqueous solutions by graphene oxide.” Journal of Hazardous Materials, vol. 282, 2015, pp. 201–207., doi:10.1016/j.jhazmat.2014.03.063.
- Zhang, Ming, et al. “Slow-Release fertilizer encapsulated by graphene oxide films.” Chemical Engineering Journal, vol. 255, 2014, pp. 107–113., doi:10.1016/j.cej.2014.06.023.
- He, Guang, et al. “Stable Cycling of a Scalable Graphene-Encapsulated Nanocomposite for Lithium–Sulfur Batteries.” ACS Applied Materials & Interfaces, vol. 6, no. 14, May 2014, pp. 10917–10923., doi:10.1021/am500632b.
- Li, Shanghao, et al. “Strong and Selective Adsorption of Lysozyme on Graphene Oxide.” ACS Applied Materials & Interfaces, vol. 6, no. 8, Aug. 2014, pp. 5704–5712., doi:10.1021/am500254e.
- Vanegas, D. C., et al. “Xanthine oxidase biosensor for monitoring meat spoilage.” Smart Biomedical and Physiological Sensor Technology XI, 2014, doi:10.1117/12.2050489.
- Nguyen, Le Huy, et al. “Functionalization of reduced graphene oxide by electroactive polymer for biosensing applications.” Advances in Natural Sciences: Nanoscience and Nanotechnology, vol. 5, no. 3, Sept. 2014, p. 035005., doi:10.1088/2043-6262/5/3/035005.
- Li, Shanghao. “Analysis of the Interactions between Graphene Oxide and Biomolecules and Protein Fibrillation Using Surface Chemistry and Spectroscopy.” University of Miami Scholarly Repository.
- Ding, Zhuhong, et al. “Filtration and transport of heavy metals in graphene oxide enabled sand columns.” Chemical Engineering Journal, vol. 257, 2014, pp. 248–252., doi:10.1016/j.cej.2014.07.034.
- Sims, Christopher M., et al. “CO tolerance of Pt and PtSn intermetallic electrocatalysts on synthetically modified reduced graphene oxide supports.” Dalton Transactions, vol. 44, no. 3, 2015, pp. 977–987., doi:10.1039/c4dt02544j.
- Sun, Yuanyuan, et al. “Transport, retention, and size perturbation of graphene oxide in saturated porous media: Effects of input concentration and grain size.” Water Research, vol. 68, 2015, pp. 24–33., doi:10.1016/j.watres.2014.09.025.
- Feng, Xuefei, et al. “Understanding the degradation mechanism of rechargeable lithium/Sulfur cells: a comprehensive study of the sulfur–graphene oxide cathode after discharge–charge cycling.” Phys. Chem., vol. 16, no. 32, 2014, pp. 16931–16940., doi:10.1039/c4cp01341g.
- Luan, Xinning, et al. “Enhanced photocatalytic activity of graphene oxide/Titania nanosheets composites for methylene blue degradation.” Materials Science in Semiconductor Processing, vol. 30, 2015, pp. 592–598., doi:10.1016/j.mssp.2014.10.032.
- Burrs, S. L. “A comparative study of graphene–hydrogel hybrid bionanocomposites for biosensing.” Analyst, no. 5, 24 Dec. 2014, doi:10.1039/C4AN01788A.
- Tangorra, Rocco Roberto, et al. “Photoactive film by covalent immobilization of a bacterial photosynthetic protein on reduced graphene oxide surface.” MRS Proceedings, vol. 1717, 2015, doi:10.1557/opl.2015.18.
- Huang, Taizhong, et al. “A high-Performance catalyst support for methanol oxidation with graphene and vanadium carbonitride.” Nanoscale, vol. 7, no. 4, 2015, pp. 1301–1307., doi:10.1039/c4nr05244g.
- Chen, Hang, and Tobin Filleter. “Effect of structure on the tribology of ultrathin graphene and graphene oxide films.” Nanotechnology, vol. 26, no. 13.
- Huynh, Vien T., et al. “Polymer coating of graphene oxide via reversible addition-Fragmentation chain transfer mediated emulsion polymerization.” Journal of Polymer Science Part A: Polymer Chemistry, vol. 53, no. 12, 2015, pp. 1413–1421., doi:10.1002/pola.27596.
- Cui, Shumao, et al. “Stabilizing MoS2Nanosheets through SnO2Nanocrystal Decoration for High-Performance Gas Sensing in Air.” Small, vol. 11, no. 19, 2015, pp. 2305–2313., doi:10.1002/smll.201402923.
- Gao, Xianfeng, et al. “A Multilayered Silicon-Reduced Graphene Oxide Electrode for High Performance Lithium-Ion Batteries.” ACS Applied Materials & Interfaces, vol. 7, no. 15, 2015, pp. 7855–7862., doi:10.1021/acsami.5b01230.
- Huang, Po-Jung Jimmy, et al. “Inhibiting the VIM-2 Metallo-β-Lactamase by Graphene Oxide and Carbon Nanotubes.” ACS Applied Materials & Interfaces, vol. 7, no. 18, 2015, pp. 9898–9903., doi:10.1021/acsami.5b01954.
- Zhao, Yingcan, and Chad T. Jafvert. “Environmental photochemistry of single layered graphene oxide in water.” Environmental Science: Nano, vol. 2, no. 2, 2015, pp. 136–142., doi:10.1039/c4en00209a.
- Liang, Xiao, et al. “A highly efficient polysulfide mediator for lithium–sulfur batteries.” Nature Communications, vol. 6, June 2015, p. 5682., doi:10.1038/ncomms6682.
- Vanegas, D. C., et al. “Rapid detection of listeria spp. using an internalin A aptasensor based on carbon-Metal nanohybrid structures.” Smart Biomedical and Physiological Sensor Technology XII, 2015, doi:10.1117/12.2177441.
- Vanegas, Diana C., et al. “A self-Referencing biosensor for real-Time monitoring of physiological ATP transport in plant systems.” Biosensors and Bioelectronics, vol. 74, 2015, pp. 37–44., doi:10.1016/j.bios.2015.05.027.
- Huang, Po-Jung Jimmy, et al. “Hg2 detection using a phosphorothioate RNA probe adsorbed on graphene oxide and a comparison with thymine-Rich DNA.” The Analyst, vol. 141, no. 12, 2016, pp. 3788–3793., doi:10.1039/c5an02031j.
- El-Amin, Ayman A., and Abdelhameed M. Othman. “Novel GO-LaSmO2 Nanocomposite as an Effective Electrode Material for Hydrogen Fuel Cells.” Jom, vol. 68, no. 4, Aug. 2015, pp. 1209–1215., doi:10.1007/s11837-015-1741-9.
- Song, Min-Kyu, et al. “Effects of cell construction parameters on the performance of lithium/Sulfur cells.” AIChE Journal, vol. 61, no. 9, July 2015, pp. 2749–2756., doi:10.1002/aic.14947.
- Dong, Shunan, et al. “Graphene oxide as filter media to remove levofloxacin and lead from aqueous solution.” Chemosphere, vol. 150, 2016, pp. 759–764., doi:10.1016/j.chemosphere.2015.11.075.
- Chang, Jingbo, et al. “Real-Time detection of mercury ions in water using a reduced graphene oxide/DNA field-Effect transistor with assistance of a passivation layer.” Sensing and Bio-Sensing Research, vol. 5, 2015, pp. 97–104., doi:10.1016/j.sbsr.2015.07.009.
- Xu, Wangwang, et al. “Hierarchical Graphene-Encapsulated Hollow SnO2@SnS2 Nanostructures with Enhanced Lithium Storage Capability.” ACS Applied Materials & Interfaces, vol. 7, no. 40, 2015, pp. 22533–22541., doi:10.1021/acsami.5b06765.
- Ye, Yifan. “X-Ray Absorption Spectroscopy Characterization of a Li/S Cell.” Nanomaterials, 2016, doi:10.3390/nano6010014.
- Nguyen, Trang H. D., et al. “Toxicity of Graphene Oxide on Intestinal Bacteria and Caco-2 Cells.” Journal of Food Protection, vol. 78, no. 5, 2015, pp. 996–1002., doi:10.4315/0362-028x.jfp-14-463.
- Huang, Po-Jung Jimmy, et al. “Liposome/Graphene Oxide Interaction Studied by Isothermal Titration Calorimetry.” Langmuir, vol. 32, no. 10, Apr. 2016, pp. 2458–2463., doi:10.1021/acs.langmuir.6b00006.
- Liu, Biwu, et al. “Graphene oxide surface blocking agents can increase the DNA biosensor sensitivity.” Biotechnology Journal, vol. 11, no. 6, Nov. 2016, pp. 780–787., doi:10.1002/biot.201500540.
- Liu, Taoze, et al. “Biochar-Supported carbon nanotube and graphene oxide nanocomposites for Pb(Ii) and Cd(Ii) removal.” RSC Advances, vol. 6, no. 29, 2016, pp. 24314–24319., doi:10.1039/c6ra01895e.
- Nguyen, T. Dung, et al. “One-Step Electrosynthesis of Poly(1,5-Diaminonaphthalene)/Graphene Nanocomposite as Platform for Lead Detection in Water.” Electroanalysis, vol. 28, no. 8, Nov. 2016, pp. 1907–1913., doi:10.1002/elan.201501075.
- Tegou, E., et al. “Low-Temperature thermal reduction of graphene oxide films in ambient atmosphere: Infra-Red spectroscopic studies and gas sensing applications.” Microelectronic Engineering, vol. 159, 2016, pp. 146–150., doi:10.1016/j.mee.2016.03.030.
- Xu, Wangwang, et al. “Direct growth of an economic green energy storage material: a monocrystalline jarosite-KFe3(SO4)2(OH)6-Nanoplates@RGO hybrid as a superior lithium-Ion battery cathode.” Journal of Materials Chemistry A, vol. 4, no. 10, 2016, pp. 3735–3742., doi:10.1039/c5ta10622b.
- Pandey, Gaind P. “Mesoporous Hybrids of Reduced Graphene Oxide and Vanadium Pentoxide for Enhanced Performance in Lithium-Ion Batteries and Electrochemical Capacitors.” Applied Materials & Interfaces, 24 Mar. 2016, pp. 9200–9210., doi:10.1021/acsami.6b02372.
- Singh, Bharti, et al. “Exploring Nanoscale Electrical Properties of CuO-Graphene Based Hybrid Interfaced Memory Device by Conductive Atomic Force Microscopy.” Journal of Nanoscience and Nanotechnology, vol. 16, no. 4, Jan. 2016, pp. 4044–4051., doi:10.1166/jnn.2016.10713.
- Chakrabarti, Barun, et al. “Performance Enhancement of Reduced Graphene Oxide-Modified Carbon Electrodes for Vanadium Redox-Flow Systems.” ChemElectroChem, vol. 4, no. 1, Mar. 2016, pp. 194–200., doi:10.1002/celc.201600402.
- Lu, Taotao, et al. “Effects of clay minerals on transport of graphene oxide in saturated porous media.” Environmental Toxicology and Chemistry, vol. 36, no. 3, 2016, pp. 655–660., doi:10.1002/etc.3605.
- Ali, Md. Azahar, et al. “Tunable bioelectrodes with wrinkled-Ridged graphene oxide surfaces for electrochemical nitrate sensors.” RSC Advances, vol. 6, no. 71, 2016, pp. 67184–67195., doi:10.1039/c6ra09621b.
- Wang, Hai-Sheng, et al. “The preparation of reduced graphene oxide and its photothermal therapy of gliomas in vivo and in vitro.” Int J Clin Exp Med, 2016.
- Gutić, Sanjin, et al. “Surface Charge Storage Properties of Selected Graphene Samples in pH-Neutral Aqueous Solutions of Alkali Metal Chlorides - Particularities and Universalities.” International Journal of Electrochemical Science, 2016, pp. 8662–8682., doi:10.20964/2016.10.47.
- Nieto, Andy, et al. “Graphene reinforced metal and ceramic matrix composites: a review.” International Materials Reviews, vol. 62, no. 5, 2016, pp. 241–302., doi:10.1080/09506608.2016.1219481.
- Mauro, Nicolò, et al. “Enhanced adhesion and in situ photothermal ablation of cancer cells in surface-Functionalized electrospun microfiber scaffold with graphene oxide.” International Journal of Pharmaceutics, vol. 526, no. 1-2, 2017, pp. 167–177., doi:10.1016/j.ijpharm.2017.04.045.
- Gatti, Teresa, et al. “A D-π-A organic dye – Reduced graphene oxide covalent dyad as a new concept photosensitizer for light harvesting applications.” Carbon, vol. 115, 2017, pp. 746–753., doi:10.1016/j.carbon.2017.01.081.
- Zhou, Yikang, et al. “Graphene-Doped polyaniline nanocomposites as electromagnetic wave absorbing materials.” Journal of Materials Science: Materials in Electronics, vol. 28, no. 15, May 2017, pp. 10921–10928., doi:10.1007/s10854-017-6872-z.
- Mauro, Nicolò, et al. “Photothermal Ablation of Cancer Cells Using Folate-Coated Gold/ Graphene Oxide Composite.” Current Drug Delivery, vol. 14, no. 3, 2017, pp. 433–443., doi:10.2174/1567201813666160520113804.
- Wang, Mei, et al. “Effects of temperature on graphene oxide deposition and transport in saturated porous media.” Journal of Hazardous Materials, vol. 331, 2017, pp. 28–35., doi:10.1016/j.jhazmat.2017.02.014.
- Katsumiti, Alberto, et al. “Intracellular localization and toxicity of graphene oxide and reduced graphene oxide nanoplatelets to mussel hemocytes in vitro.” Aquatic Toxicology, vol. 188, 2017, pp. 138–147., doi:10.1016/j.aquatox.2017.04.016.
- Nath, A., et al. “Universal conformal ultrathin dielectrics on epitaxial graphene enabled by a graphene oxide seed layer.” Applied Physics Letters, vol. 110, no. 1, Apr. 2017, p. 013106., doi:10.1063/1.4973200.
- Fiorica, Calogero, et al. “Double-Network-Structured Graphene Oxide-Containing Nanogels as Photothermal Agents for the Treatment of Colorectal Cancer.” Biomacromolecules, vol. 18, no. 3, 2017, pp. 1010–1018., doi:10.1021/acs.biomac.6b01897.
- Shirai, Akihiro, et al. “Development of a single-Step immunoassay microdevice based on a graphene oxide-Containing hydrogel possessing fluorescence quenching and size separation functions.” The Analyst, vol. 142, no. 3, 2017, pp. 472–477., doi:10.1039/c6an02485h.
- Zhao, Yingcan, et al. “Light-Independent redox reactions of graphene oxide in water: Electron transfer from NADH through graphene oxide to molecular oxygen, producing reactive oxygen species.” Carbon, vol. 123, 2017, pp. 216–222., doi:10.1016/j.carbon.2017.07.048.
- Mauro, Nicolò, et al. “Enhanced adhesion and in situ photothermal ablation of cancer cells in surface-Functionalized electrospun microfiber scaffold with graphene oxide.” International Journal of Pharmaceutics, vol. 526, no. 1-2, 2017, pp. 167–177., doi:10.1016/j.ijpharm.2017.04.045.
- Wang, Yong, and Long Jiang. “Roles of Graphene Oxide in Hydrothermal Carbonization and Microwave Irradiation of Distiller’s Dried Grains with Solubles To Produce Supercapacitor Electrodes.” ACS Sustainable Chemistry & Engineering, vol. 5, no. 6, May 2017, pp. 5588–5597., doi:10.1021/acssuschemeng.7b01074.
- Wang, Mei, et al. “Effects of temperature on graphene oxide deposition and transport in saturated porous media.” Journal of Hazardous Materials, vol. 331, 2017, pp. 28–35., doi:10.1016/j.jhazmat.2017.02.014.
- Katsumiti, Alberto, et al. “Intracellular localization and toxicity of graphene oxide and reduced graphene oxide nanoplatelets to mussel hemocytes in vitro.” Aquatic Toxicology, vol. 188, 2017, pp. 138–147., doi:10.1016/j.aquatox.2017.04.016.
- Gutić, Sanjin J., et al. “Improved catalysts for hydrogen evolution reaction in alkaline solutions through the electrochemical formation of nickel-Reduced graphene oxide interface.” Physical Chemistry, vol. 19, no. 20, 2017, pp. 13281–13293., doi:10.1039/c7cp01237c.
- Jernigan, Glenn G., et al. “Physical properties of nanometer graphene oxide films partially and fully reduced by annealing in ultra-High vacuum.” Journal of Applied Physics, vol. 122, no. 7, 2017, p. 075301., doi:10.1063/1.4998812.
- De Marco, Martina, et al. “Hybrid effects in graphene oxide/Carbon nanotube-Supported layered double hydroxides: enhancing the CO2 sorption properties.” Carbon, vol. 123, Oct. 2017, doi:10.1016/j.carbon.2017.07.094.
- Hwa, Yoon, et al. “Aqueous-Processable Redox-Active Supramolecular Polymer Binders for Advanced Lithium/Sulfur Cells.” Chemistry of Materials, 2018, doi:10.1021/acs.chemmater.7b03870.
- Mao, Shun, et al. “Ultrasensitive detection of orthophosphate ions with reduced graphene oxide/Ferritin field-Effect transistor sensors.” Environmental Science: Nano, vol. 4, no. 4, 2017, pp. 856–863., doi:10.1039/c6en00661b.
- Maity, Arnab, et al. “Pulse-Driven Capacitive Lead Ion Detection with Reduced Graphene Oxide Field-Effect Transistor Integrated with an Analyzing Device for Rapid Water Quality Monitoring.” ACS Sensors, vol. 2, no. 11, 2017, pp. 1653–1661., doi:10.1021/acssensors.7b00496.
- Ben-David, Jonathon, et al. “Poly(3,4-Ethylenedioxythiophene):Polystyrene sulfonate-Free silver nanowire/Single walled carbon nanotube transparent electrodes using graphene oxide.” Thin Solid Films, vol. 616, 2016, pp. 515–520., doi:10.1016/j.tsf.2016.09.014.
- Ratajczak, Katarzyna, and Magdalena Stobiecka. “Ternary Interactions and Energy Transfer between Fluorescein Isothiocyanate, Adenosine Triphosphate, and Graphene Oxide Nanocarriers.” The Journal of Physical Chemistry B, vol. 121, no. 28, May 2017, pp. 6822–6830., doi:10.1021/acs.jpcb.7b04295.
- Ha, Dat Thinh. "Developing a New Sensing Technology for Double-Stranded DNA Detection Utilizing Engineered Zinc Finger Proteins and Nanomaterials." (2018).
- Zangmeister, Christopher D., Rian You, Elizabeth M. Lunny, Arne E. Jacobson, Mitchio Okumura, Michael R. Zachariah, and James G. Radney. "Measured in-situ mass absorption spectra for nine forms of highly-absorbing carbonaceous aerosol." Carbon 136 (2018): 85-93.
- Li, Zeyang. "Development of VHH-and antibody-based imaging and diagnostic tools." PhD diss., Massachusetts Institute of Technology, 2018.
- Choi, Hayelin, Phuong Thi Nguyen, and Jung Bin In. "Laser transmission welding and surface modification of graphene film for flexible supercapacitor applications." Applied Surface Science (2019).
- Park, Jihyeon, Sudeok Kim, Gibaek Lee, and Jinsub Choi. "RGO-Coated TiO2 Microcones for High-Rate Lithium-Ion Batteries." ACS Omega 3, no. 8 (2018): 10205-10210.
|
-
FAQ
How do I disperse single layer Graphene Oxide into a suitable carrier?
For dispersion in water 1) Use distilled or deionized water 2) Add graphene powder 3) Use sonication power of 200W for about 30mins (adjust it based on your application)
For dispersion in ethanol 1) Use pure ethanol 2) Add graphene powder 3) Use sonication power of 200W for about 30mins (adjust it based on your application)
Is Graphene Oxide fully oxidized? What is the C:O ratio?
Graphene Oxide (GO) is produced by the classic modified Hummer’s method‚ which yields an oxidization level of ≥95%. Regarding the C:O ratio‚ there is some variance inherent in the manufacturing process but the figures below give you an approximation of what to expect (please use the number in the table as a reference number):
Sample |
N (wt %) |
C (wt %) |
O (wt %) |
C/O at. ratio |
Graphene Oxide |
0 |
51.26 |
40.78 |
1.67 |
Research Citations of ACS Material Products
- Mukherjee, Rahul, et al. “Photothermally Reduced Graphene as High-Power Anodes for Lithium-Ion Batteries.” ACS Nano, vol. 6, no. 9, 2012, pp. 7867–7878., doi:10.1021/nn303145j.
- Chow, Philippe K., et al. “Mechanical Property Enhancement of Layered Reduced Graphene Oxide Papers by Non-Covalent Modification with Terephthalic Acid.” Particle & Particle Systems Characterization, vol. 31, no. 3, 2013, pp. 337–341., doi:10.1002/ppsc.201300206.
- Feng, Xuefei, et al. “Understanding the degradation mechanism of rechargeable lithium/Sulfur cells: a comprehensive study of the sulfur–graphene oxide cathode after discharge–charge cycling.” Phys. Chem., vol. 16, no. 32, 2014, pp. 16931–16940., doi:10.1039/c4cp01341g.
- Cui, Shumao, et al. “Ultrasensitive Chemical Sensing through Facile Tuning Defects and Functional Groups in Reduced Graphene Oxide.” Analytical Chemistry, vol. 86, no. 15, Nov. 2014, pp. 7516–7522., doi:10.1021/ac501274z.
- Mukherjee, Rahul, et al. “Defect-Induced plating of lithium metal within porous graphene networks.” Nature Communications, vol. 5, 2014, doi:10.1038/ncomms4710.
- Lammel, Tobias, et al. “Internalization and cytotoxicity of graphene oxide and carboxyl graphene nanoplatelets in the human hepatocellular carcinoma cell line Hep G2.” Particle and Fibre Toxicology, vol. 10, no. 1, 2013, p. 27., doi:10.1186/1743-8977-10-27.
- Cheng, Jianli, et al. “Self-Assembled V2O5 nanosheets/Reduced graphene oxide hierarchical nanocomposite as a high-Performance cathode material for lithium ion batteries.” Journal of Materials Chemistry A, vol. 1, no. 36, 2013, p. 10814., doi:10.1039/c3ta12066j.
- Li, Shanghao, et al. “Interaction between Graphene Oxide and Pluronic F127 at the Air–Water Interface.” Langmuir, vol. 29, no. 19, Feb. 2013, pp. 5742–5748., doi:10.1021/la401056t.
- Othman, Abdelhameed M., et al. “Enhancing selectivity in spectrofluorimetric determination of tryptophan by using graphene oxide nanosheets.” Analytica Chimica Acta, vol. 787, 2013, pp. 226–232., doi:10.1016/j.aca.2013.05.036.
- Zhang, Xu, et al. “Effects of Polyethylene Glycol on DNA Adsorption and Hybridization on Gold Nanoparticles and Graphene Oxide.” Langmuir, vol. 28, no. 40, 2012, pp. 14330–14337., doi:10.1021/la302799s.
- Li, Shanghao, et al. “Graphene Oxide as a Quencher for Fluorescent Assay of Amino Acids, Peptides, and Proteins.” ACS Applied Materials & Interfaces, vol. 4, no. 12, Mar. 2012, pp. 7069–7075., doi:10.1021/am302704a.
- Ip, Alexander C.-F., et al. “Oxidation Level-Dependent Zwitterionic Liposome Adsorption and Rupture by Graphene-Based Materials and Light-Induced Content Release.” Small, vol. 9, no. 7, 2012, pp. 1030–1035., doi:10.1002/smll.201202710.
- Chang, Jingbo, et al. “Ultrasonic-Assisted self-Assembly of monolayer graphene oxide for rapid detection of Escherichia coli bacteria.” Nanoscale, vol. 5, no. 9, 2013, p. 3620., doi:10.1039/c3nr00141e.
- Liu, Lin, et al. “Deposition and transport of graphene oxide in saturated and unsaturated porous media.” Chemical Engineering Journal, vol. 229, 1 Aug. 2013, pp. 444–449., doi:10.1016/j.cej.2013.06.030.
- Li, Shanghao, et al. “Head Groups of Lipids Govern the Interaction and Orientation between Graphene Oxide and Lipids.” The Journal of Physical Chemistry C, vol. 117, no. 31, 2013, pp. 16150–16158., doi:10.1021/jp405991q.
- Tran, H. V., et al. “Antibodies Directed to RNA/DNA Hybrids: An Electrochemical Immunosensor for MicroRNAs Detection using Graphene-Composite Electrodes.” Analytical Chemistry, vol. 85, no. 17, 2013, pp. 8469–8474., doi:10.1021/ac402154z.
- Liu, Biwu, et al. “Mechanisms of DNA Sensing on Graphene Oxide.” Analytical Chemistry, vol. 85, no. 16, June 2013, pp. 7987–7993., doi:10.1021/ac401845p.
- Luan, Xinning, et al. “Electrophoretic deposition of reduced graphene oxide nanosheets on TiO2 nanotube arrays for dye-Sensitized solar cells.” Electrochimica Acta, vol. 111, 2013, pp. 216–222., doi:10.1016/j.electacta.2013.08.016.
- Zhang, Ming, et al. “Synthesis of a multifunctional graphene–carbon nanotube aerogel and its strong adsorption of lead from aqueous solution.” RSC Advances, vol. 3, no. 43, 2013, p. 21099., doi:10.1039/c3ra44340j.
- Wu, Lei, et al. “Aggregation Kinetics of Graphene Oxides in Aqueous Solutions: Experiments, Mechanisms, and Modeling.” Langmuir, vol. 29, no. 49, Mar. 2013, pp. 15174–15181., doi:10.1021/la404134x.
- Wang, Feng, and Juewen Liu. “Nanodiamond decorated liposomes as highly biocompatible delivery vehicles and a comparison with carbon nanotubes and graphene oxide.” Nanoscale, vol. 5, no. 24, 2013, p. 12375., doi:10.1039/c3nr04143c.
- Zhang, Ming, et al. “Simple approach for large-Scale production of reduced graphene oxide films.” Chemical Engineering Journal, vol. 243, 2014, pp. 340–346., doi:10.1016/j.cej.2014.01.019.
- Frechette, M. F., et al. “Preparation and dielectric responses of solid epoxy composites containing a mixture of epoxy powder ball-Milled with GO.” 2013 Annual Report Conference on Electrical Insulation and Dielectric Phenomena, 2013, doi:10.1109/ceidp.2013.6748335.
- Chaturvedi, P. et al. “A nanoceria–platinum–graphene nanocomposite for electrochemical biosensing.” Biosensors and Bioelectronics, vol. 58, 15 Aug. 2014, doi:10.1016/j.bios.2014.02.021.
- Lammel, Tobias, and José M. Navas. “Graphene nanoplatelets spontaneously translocate into the cytosol and physically interact with cellular organelles in the fish cell line PLHC-1.” Aquatic Toxicology, vol. 150, 2014, pp. 55–65., doi:10.1016/j.aquatox.2014.02.016.
- Souza, Camille De, et al. “Electrocatalytic miRNA Detection Using Cobalt Porphyrin-Modified Reduced Graphene Oxide.” Sensors, vol. 14, no. 6, June 2014, pp. 9984–9994., doi:10.3390/s140609984.
- Lammel, Tobias, et al. “Potentiating effect of graphene nanomaterials on aromatic environmental pollutant-Induced cytochrome P450 1A expression in the topminnow fish hepatoma cell line PLHC-1.” Environmental Toxicology, vol. 30, no. 10, May 2014, pp. 1192–1204., doi:10.1002/tox.21991.
- Tran, H.V., et al. “An electrochemical ELISA-like immunosensor for miRNAs detection based on screen-Printed gold electrodes modified with reduced graphene oxide and carbon nanotubes.” Biosensors and Bioelectronics, vol. 62, 2014, pp. 25–30., doi:10.1016/j.bios.2014.06.014.
- Wang, Feng, and Juewen Liu. “Platinated DNA oligonucleotides: new probes forming ultrastable conjugates with graphene oxide.” Nanoscale, vol. 6, no. 12, 2014, p. 7079., doi:10.1039/c4nr00867g.
- Chen, Hao, et al. “Removal of sulfamethoxazole and ciprofloxacin from aqueous solutions by graphene oxide.” Journal of Hazardous Materials, vol. 282, 2015, pp. 201–207., doi:10.1016/j.jhazmat.2014.03.063.
- Zhang, Ming, et al. “Slow-Release fertilizer encapsulated by graphene oxide films.” Chemical Engineering Journal, vol. 255, 2014, pp. 107–113., doi:10.1016/j.cej.2014.06.023.
- He, Guang, et al. “Stable Cycling of a Scalable Graphene-Encapsulated Nanocomposite for Lithium–Sulfur Batteries.” ACS Applied Materials & Interfaces, vol. 6, no. 14, May 2014, pp. 10917–10923., doi:10.1021/am500632b.
- Li, Shanghao, et al. “Strong and Selective Adsorption of Lysozyme on Graphene Oxide.” ACS Applied Materials & Interfaces, vol. 6, no. 8, Aug. 2014, pp. 5704–5712., doi:10.1021/am500254e.
- Vanegas, D. C., et al. “Xanthine oxidase biosensor for monitoring meat spoilage.” Smart Biomedical and Physiological Sensor Technology XI, 2014, doi:10.1117/12.2050489.
- Nguyen, Le Huy, et al. “Functionalization of reduced graphene oxide by electroactive polymer for biosensing applications.” Advances in Natural Sciences: Nanoscience and Nanotechnology, vol. 5, no. 3, Sept. 2014, p. 035005., doi:10.1088/2043-6262/5/3/035005.
- Li, Shanghao. “Analysis of the Interactions between Graphene Oxide and Biomolecules and Protein Fibrillation Using Surface Chemistry and Spectroscopy.” University of Miami Scholarly Repository.
- Ding, Zhuhong, et al. “Filtration and transport of heavy metals in graphene oxide enabled sand columns.” Chemical Engineering Journal, vol. 257, 2014, pp. 248–252., doi:10.1016/j.cej.2014.07.034.
- Sims, Christopher M., et al. “CO tolerance of Pt and PtSn intermetallic electrocatalysts on synthetically modified reduced graphene oxide supports.” Dalton Transactions, vol. 44, no. 3, 2015, pp. 977–987., doi:10.1039/c4dt02544j.
- Sun, Yuanyuan, et al. “Transport, retention, and size perturbation of graphene oxide in saturated porous media: Effects of input concentration and grain size.” Water Research, vol. 68, 2015, pp. 24–33., doi:10.1016/j.watres.2014.09.025.
- Feng, Xuefei, et al. “Understanding the degradation mechanism of rechargeable lithium/Sulfur cells: a comprehensive study of the sulfur–graphene oxide cathode after discharge–charge cycling.” Phys. Chem., vol. 16, no. 32, 2014, pp. 16931–16940., doi:10.1039/c4cp01341g.
- Luan, Xinning, et al. “Enhanced photocatalytic activity of graphene oxide/Titania nanosheets composites for methylene blue degradation.” Materials Science in Semiconductor Processing, vol. 30, 2015, pp. 592–598., doi:10.1016/j.mssp.2014.10.032.
- Burrs, S. L. “A comparative study of graphene–hydrogel hybrid bionanocomposites for biosensing.” Analyst, no. 5, 24 Dec. 2014, doi:10.1039/C4AN01788A.
- Tangorra, Rocco Roberto, et al. “Photoactive film by covalent immobilization of a bacterial photosynthetic protein on reduced graphene oxide surface.” MRS Proceedings, vol. 1717, 2015, doi:10.1557/opl.2015.18.
- Huang, Taizhong, et al. “A high-Performance catalyst support for methanol oxidation with graphene and vanadium carbonitride.” Nanoscale, vol. 7, no. 4, 2015, pp. 1301–1307., doi:10.1039/c4nr05244g.
- Chen, Hang, and Tobin Filleter. “Effect of structure on the tribology of ultrathin graphene and graphene oxide films.” Nanotechnology, vol. 26, no. 13.
- Huynh, Vien T., et al. “Polymer coating of graphene oxide via reversible addition-Fragmentation chain transfer mediated emulsion polymerization.” Journal of Polymer Science Part A: Polymer Chemistry, vol. 53, no. 12, 2015, pp. 1413–1421., doi:10.1002/pola.27596.
- Cui, Shumao, et al. “Stabilizing MoS2Nanosheets through SnO2Nanocrystal Decoration for High-Performance Gas Sensing in Air.” Small, vol. 11, no. 19, 2015, pp. 2305–2313., doi:10.1002/smll.201402923.
- Gao, Xianfeng, et al. “A Multilayered Silicon-Reduced Graphene Oxide Electrode for High Performance Lithium-Ion Batteries.” ACS Applied Materials & Interfaces, vol. 7, no. 15, 2015, pp. 7855–7862., doi:10.1021/acsami.5b01230.
- Huang, Po-Jung Jimmy, et al. “Inhibiting the VIM-2 Metallo-β-Lactamase by Graphene Oxide and Carbon Nanotubes.” ACS Applied Materials & Interfaces, vol. 7, no. 18, 2015, pp. 9898–9903., doi:10.1021/acsami.5b01954.
- Zhao, Yingcan, and Chad T. Jafvert. “Environmental photochemistry of single layered graphene oxide in water.” Environmental Science: Nano, vol. 2, no. 2, 2015, pp. 136–142., doi:10.1039/c4en00209a.
- Liang, Xiao, et al. “A highly efficient polysulfide mediator for lithium–sulfur batteries.” Nature Communications, vol. 6, June 2015, p. 5682., doi:10.1038/ncomms6682.
- Vanegas, D. C., et al. “Rapid detection of listeria spp. using an internalin A aptasensor based on carbon-Metal nanohybrid structures.” Smart Biomedical and Physiological Sensor Technology XII, 2015, doi:10.1117/12.2177441.
- Vanegas, Diana C., et al. “A self-Referencing biosensor for real-Time monitoring of physiological ATP transport in plant systems.” Biosensors and Bioelectronics, vol. 74, 2015, pp. 37–44., doi:10.1016/j.bios.2015.05.027.
- Huang, Po-Jung Jimmy, et al. “Hg2 detection using a phosphorothioate RNA probe adsorbed on graphene oxide and a comparison with thymine-Rich DNA.” The Analyst, vol. 141, no. 12, 2016, pp. 3788–3793., doi:10.1039/c5an02031j.
- El-Amin, Ayman A., and Abdelhameed M. Othman. “Novel GO-LaSmO2 Nanocomposite as an Effective Electrode Material for Hydrogen Fuel Cells.” Jom, vol. 68, no. 4, Aug. 2015, pp. 1209–1215., doi:10.1007/s11837-015-1741-9.
- Song, Min-Kyu, et al. “Effects of cell construction parameters on the performance of lithium/Sulfur cells.” AIChE Journal, vol. 61, no. 9, July 2015, pp. 2749–2756., doi:10.1002/aic.14947.
- Dong, Shunan, et al. “Graphene oxide as filter media to remove levofloxacin and lead from aqueous solution.” Chemosphere, vol. 150, 2016, pp. 759–764., doi:10.1016/j.chemosphere.2015.11.075.
- Chang, Jingbo, et al. “Real-Time detection of mercury ions in water using a reduced graphene oxide/DNA field-Effect transistor with assistance of a passivation layer.” Sensing and Bio-Sensing Research, vol. 5, 2015, pp. 97–104., doi:10.1016/j.sbsr.2015.07.009.
- Xu, Wangwang, et al. “Hierarchical Graphene-Encapsulated Hollow SnO2@SnS2 Nanostructures with Enhanced Lithium Storage Capability.” ACS Applied Materials & Interfaces, vol. 7, no. 40, 2015, pp. 22533–22541., doi:10.1021/acsami.5b06765.
- Ye, Yifan. “X-Ray Absorption Spectroscopy Characterization of a Li/S Cell.” Nanomaterials, 2016, doi:10.3390/nano6010014.
- Nguyen, Trang H. D., et al. “Toxicity of Graphene Oxide on Intestinal Bacteria and Caco-2 Cells.” Journal of Food Protection, vol. 78, no. 5, 2015, pp. 996–1002., doi:10.4315/0362-028x.jfp-14-463.
- Huang, Po-Jung Jimmy, et al. “Liposome/Graphene Oxide Interaction Studied by Isothermal Titration Calorimetry.” Langmuir, vol. 32, no. 10, Apr. 2016, pp. 2458–2463., doi:10.1021/acs.langmuir.6b00006.
- Liu, Biwu, et al. “Graphene oxide surface blocking agents can increase the DNA biosensor sensitivity.” Biotechnology Journal, vol. 11, no. 6, Nov. 2016, pp. 780–787., doi:10.1002/biot.201500540.
- Liu, Taoze, et al. “Biochar-Supported carbon nanotube and graphene oxide nanocomposites for Pb(Ii) and Cd(Ii) removal.” RSC Advances, vol. 6, no. 29, 2016, pp. 24314–24319., doi:10.1039/c6ra01895e.
- Nguyen, T. Dung, et al. “One-Step Electrosynthesis of Poly(1,5-Diaminonaphthalene)/Graphene Nanocomposite as Platform for Lead Detection in Water.” Electroanalysis, vol. 28, no. 8, Nov. 2016, pp. 1907–1913., doi:10.1002/elan.201501075.
- Tegou, E., et al. “Low-Temperature thermal reduction of graphene oxide films in ambient atmosphere: Infra-Red spectroscopic studies and gas sensing applications.” Microelectronic Engineering, vol. 159, 2016, pp. 146–150., doi:10.1016/j.mee.2016.03.030.
- Xu, Wangwang, et al. “Direct growth of an economic green energy storage material: a monocrystalline jarosite-KFe3(SO4)2(OH)6-Nanoplates@RGO hybrid as a superior lithium-Ion battery cathode.” Journal of Materials Chemistry A, vol. 4, no. 10, 2016, pp. 3735–3742., doi:10.1039/c5ta10622b.
- Pandey, Gaind P. “Mesoporous Hybrids of Reduced Graphene Oxide and Vanadium Pentoxide for Enhanced Performance in Lithium-Ion Batteries and Electrochemical Capacitors.” Applied Materials & Interfaces, 24 Mar. 2016, pp. 9200–9210., doi:10.1021/acsami.6b02372.
- Dong, Shunan, et al. “Retention and Release of Graphene Oxide in Structured Heterogeneous Porous Media under Saturated and Unsaturated Conditions.” Environmental Science & Technology, vol. 50, no. 19, 2016, pp. 10397–10405., doi:10.1021/acs.est.6b01948.
- Frischmann, Peter D., et al. “Redox-Active Supramolecular Polymer Binders for Lithium–Sulfur Batteries That Adapt Their Transport Properties in Operando.” Chemistry of Materials, vol. 28, no. 20, Apr. 2016, pp. 7414–7421., doi:10.1021/acs.chemmater.6b03013.
- Ferrer, Diana Iruretagoyena. “Layered Double Hydroxides Supported on Graphene Oxide for CO2 Adsorption.” Supported Layered Double Hydroxides as CO2 Adsorbents for Sorption-Enhanced H2 Production Springer Theses, 2016, pp. 85–113., doi:10.1007/978-3-319-41276-4_5.
- Singh, Bharti, et al. “Exploring Nanoscale Electrical Properties of CuO-Graphene Based Hybrid Interfaced Memory Device by Conductive Atomic Force Microscopy.” Journal of Nanoscience and Nanotechnology, vol. 16, no. 4, Jan. 2016, pp. 4044–4051., doi:10.1166/jnn.2016.10713.
- Chakrabarti, Barun, et al. “Performance Enhancement of Reduced Graphene Oxide-Modified Carbon Electrodes for Vanadium Redox-Flow Systems.” ChemElectroChem, vol. 4, no. 1, Mar. 2016, pp. 194–200., doi:10.1002/celc.201600402.
- Lu, Taotao, et al. “Effects of clay minerals on transport of graphene oxide in saturated porous media.” Environmental Toxicology and Chemistry, vol. 36, no. 3, 2016, pp. 655–660., doi:10.1002/etc.3605.
- Ali, Md. Azahar, et al. “Tunable bioelectrodes with wrinkled-Ridged graphene oxide surfaces for electrochemical nitrate sensors.” RSC Advances, vol. 6, no. 71, 2016, pp. 67184–67195., doi:10.1039/c6ra09621b.
- Wang, Hai-Sheng, et al. “The preparation of reduced graphene oxide and its photothermal therapy of gliomas in vivo and in vitro.” Int J Clin Exp Med, 2016.
- Gutić, Sanjin, et al. “Surface Charge Storage Properties of Selected Graphene Samples in pH-Neutral Aqueous Solutions of Alkali Metal Chlorides - Particularities and Universalities.” International Journal of Electrochemical Science, 2016, pp. 8662–8682., doi:10.20964/2016.10.47.
- Nieto, Andy, et al. “Graphene reinforced metal and ceramic matrix composites: a review.” International Materials Reviews, vol. 62, no. 5, 2016, pp. 241–302., doi:10.1080/09506608.2016.1219481.
- Mauro, Nicolò, et al. “Enhanced adhesion and in situ photothermal ablation of cancer cells in surface-Functionalized electrospun microfiber scaffold with graphene oxide.” International Journal of Pharmaceutics, vol. 526, no. 1-2, 2017, pp. 167–177., doi:10.1016/j.ijpharm.2017.04.045.
- Gatti, Teresa, et al. “A D-π-A organic dye – Reduced graphene oxide covalent dyad as a new concept photosensitizer for light harvesting applications.” Carbon, vol. 115, 2017, pp. 746–753., doi:10.1016/j.carbon.2017.01.081.
- Zhou, Yikang, et al. “Graphene-Doped polyaniline nanocomposites as electromagnetic wave absorbing materials.” Journal of Materials Science: Materials in Electronics, vol. 28, no. 15, May 2017, pp. 10921–10928., doi:10.1007/s10854-017-6872-z.
- Mauro, Nicolò, et al. “Photothermal Ablation of Cancer Cells Using Folate-Coated Gold/ Graphene Oxide Composite.” Current Drug Delivery, vol. 14, no. 3, 2017, pp. 433–443., doi:10.2174/1567201813666160520113804.
- Wang, Mei, et al. “Effects of temperature on graphene oxide deposition and transport in saturated porous media.” Journal of Hazardous Materials, vol. 331, 2017, pp. 28–35., doi:10.1016/j.jhazmat.2017.02.014.
- Katsumiti, Alberto, et al. “Intracellular localization and toxicity of graphene oxide and reduced graphene oxide nanoplatelets to mussel hemocytes in vitro.” Aquatic Toxicology, vol. 188, 2017, pp. 138–147., doi:10.1016/j.aquatox.2017.04.016.
- Nath, A., et al. “Universal conformal ultrathin dielectrics on epitaxial graphene enabled by a graphene oxide seed layer.” Applied Physics Letters, vol. 110, no. 1, Apr. 2017, p. 013106., doi:10.1063/1.4973200.
- Dong, Shunan, et al. “Retention and transport of graphene oxide in water-Saturated limestone media.” Chemosphere, vol. 180, Aug. 2017, pp. 506–512., doi:10.1016/j.chemosphere.2017.04.052.
- Das, Suprem R., et al. “Stem Cell Differentiation: Electrical Differentiation of Mesenchymal Stem Cells into Schwann-Cell-Like Phenotypes Using Inkjet-Printed Graphene Circuits (Adv. Healthcare Mater. 7/2017).” Advanced Healthcare Materials, vol. 6, no. 7, 2017, doi:10.1002/adhm.201770032.
- Fiorica, Calogero, et al. “Double-Network-Structured Graphene Oxide-Containing Nanogels as Photothermal Agents for the Treatment of Colorectal Cancer.” Biomacromolecules, vol. 18, no. 3, 2017, pp. 1010–1018., doi:10.1021/acs.biomac.6b01897.
- Shirai, Akihiro, et al. “Development of a single-Step immunoassay microdevice based on a graphene oxide-Containing hydrogel possessing fluorescence quenching and size separation functions.” The Analyst, vol. 142, no. 3, 2017, pp. 472–477., doi:10.1039/c6an02485h.
- Zhao, Yingcan, et al. “Light-Independent redox reactions of graphene oxide in water: Electron transfer from NADH through graphene oxide to molecular oxygen, producing reactive oxygen species.” Carbon, vol. 123, 2017, pp. 216–222., doi:10.1016/j.carbon.2017.07.048.
- Mauro, Nicolò, et al. “Enhanced adhesion and in situ photothermal ablation of cancer cells in surface-Functionalized electrospun microfiber scaffold with graphene oxide.” International Journal of Pharmaceutics, vol. 526, no. 1-2, 2017, pp. 167–177., doi:10.1016/j.ijpharm.2017.04.045.
- Wang, Yong, and Long Jiang. “Roles of Graphene Oxide in Hydrothermal Carbonization and Microwave Irradiation of Distiller’s Dried Grains with Solubles To Produce Supercapacitor Electrodes.” ACS Sustainable Chemistry & Engineering, vol. 5, no. 6, May 2017, pp. 5588–5597., doi:10.1021/acssuschemeng.7b01074.
- Wang, Mei, et al. “Effects of temperature on graphene oxide deposition and transport in saturated porous media.” Journal of Hazardous Materials, vol. 331, 2017, pp. 28–35., doi:10.1016/j.jhazmat.2017.02.014.
- Katsumiti, Alberto, et al. “Intracellular localization and toxicity of graphene oxide and reduced graphene oxide nanoplatelets to mussel hemocytes in vitro.” Aquatic Toxicology, vol. 188, 2017, pp. 138–147., doi:10.1016/j.aquatox.2017.04.016.
- Samanidou, Victoria, et al. “Sol-Gel-Graphene-Based fabric-Phase sorptive extraction for cow and human breast milk sample cleanup for screening bisphenol A and residual dental restorative material before analysis by HPLC with diode array detection.” Journal of Separation Science, vol. 40, no. 12, 2017, pp. 2612–2619., doi:10.1002/jssc.201700256.
- Gutić, Sanjin J., et al. “Improved catalysts for hydrogen evolution reaction in alkaline solutions through the electrochemical formation of nickel-Reduced graphene oxide interface.” Physical Chemistry, vol. 19, no. 20, 2017, pp. 13281–13293., doi:10.1039/c7cp01237c.
- Jernigan, Glenn G., et al. “Physical properties of nanometer graphene oxide films partially and fully reduced by annealing in ultra-High vacuum.” Journal of Applied Physics, vol. 122, no. 7, 2017, p. 075301., doi:10.1063/1.4998812.
- Nassef, Hossam, et al. “Uptake of Tyrosine Amino Acid on Nano-Graphene Oxide.” Materials, vol. 11, no. 1, Apr. 2018, p. 68., doi:10.3390/ma11010068.
- Huang, Po-Jung Jimmy, et al. “Liposome/Graphene Oxide Interaction Studied by Isothermal Titration Calorimetry.” Langmuir, vol. 32, no. 10, Apr. 2016, pp. 2458–2463., doi:10.1021/acs.langmuir.6b00006.
- Liu, Biwu, et al. “Graphene oxide surface blocking agents can increase the DNA biosensor sensitivity.” Biotechnology Journal, vol. 11, no. 6, Nov. 2016, pp. 780–787., doi:10.1002/biot.201500540.
- Wang, Mei, et al. “Effects of temperature on aggregation kinetics of graphene oxide in aqueous solutions.” Colloids and Surfaces A: Physicochemical and Engineering Aspects, vol. 538, 2018, pp. 63–72., doi:10.1016/j.colsurfa.2017.10.061.
- Sun, Kaixuan, et al. “Graphene oxide-Facilitated transport of levofloxacin and ciprofloxacin in saturated and unsaturated porous media.” Journal of Hazardous Materials, vol. 348, 2018, pp. 92–99., doi:10.1016/j.jhazmat.2018.01.032.
- Carroll, Benjamin O. . “Analysis of graphite oxide and graphene as enhancers for NATO F-76 diesel fuel.” June 2015.
- Chen, Junhong, and Jingbo Chang. Real-Time detection of water contaminants.
- Seehra, Mohindar S., et al. “Correlation between X-Ray diffraction and Raman spectra of 16 commercial graphene–based materials and their resulting classification.” Carbon, vol. 111, 2017, pp. 380–385., doi:10.1016/j.carbon.2016.10.010.
- Stanković, Dalibor M., et al. “Design of titanium nitride- and wolfram carbide-Doped RGO/GC electrodes for determination of gallic acid.” Analytical Biochemistry, vol. 539, 2017, pp. 104–112., doi:10.1016/j.ab.2017.10.018.
- Cho, Young Hoon, et al. “Water and ion sorption, diffusion, and transport in graphene oxide membranes revisited.” Journal of Membrane Science, vol. 544, 2017, pp. 425–435., doi:10.1016/j.memsci.2017.09.043.
- Pang, Quan, et al. “A Comprehensive Approach toward Stable Lithium-Sulfur Batteries with High Volumetric Energy Density.” Advanced Energy Materials, vol. 7, no. 6, 2016, p. 1601630., doi:10.1002/aenm.201601630.
- Chaturvedi, P., et al. “Microprofiling real time nitric oxide flux for field studies using a stratified nanohybrid carbon–metal electrode.” Analytical Methods, vol. 9, no. 42, 2017, pp. 6061–6072., doi:10.1039/c7ay01964e.
- Wang, Yan, and Leanne M. Gilbertson. “Informing rational design of graphene oxide through surface chemistry manipulations: properties governing electrochemical and biological activities.” Green Chemistry, vol. 19, no. 12, 2017, pp. 2826–2838., doi:10.1039/c7gc00159b.
- Cho, Eun Seon, et al. “Hierarchically Controlled Inside-Out Doping of Mg Nanocomposites for Moderate Temperature Hydrogen Storage.” Advanced Functional Materials, vol. 27, no. 47, 2017, p. 1704316., doi:10.1002/adfm.201704316.
- Gabrielli, Luca, et al. “Controlling the Decoration of the Reduced Graphene Oxide Surface with Pyrene-Functionalized Gold Nanoparticles.” Physica status solidi (b), vol. 254, no. 11, 2017, p. 1700281., doi:10.1002/pssb.201700281.
- Wang, Mei, et al. “Concurrent aggregation and transport of graphene oxide in saturated porous media: Roles of temperature, cation type, and electrolyte concentration.” Environmental Pollution, vol. 235, 2018, pp. 350–357., doi:10.1016/j.envpol.2017.12.063.
- Kahyaoglu, Leyla Nesrin, and Jenna L. Rickus. "Robust Covalent Coupling Scheme for the Development of FRET Aptasensor based on Amino-Silane-Modified Graphene Oxide." Langmuir 34, no. 48 (2018): 14586-14596.
- Lazarević-Pašti, Tamara, Vladan Anićijević, Miloš Baljozović, Dragana Vasić Anićijević, Sanjin Gutić, Vesna Vasić, Natalia V. Skorodumova, and Igor A. Pašti. "The impact of the structure of graphene-based materials on the removal of organophosphorus pesticides from water." Environmental Science: Nano (2018).
- Gao, Xianfeng, Fenfen Wang, Sam Gollon, and Chis Yuan. "Micro Silicon-Graphene-CNT anode for full cell lithium ion battery." (2018).
- Wang, Mei, Bin Gao, Deshan Tang, and Congrong Yu. "Concurrent aggregation and transport of graphene oxide in saturated porous media: roles of temperature, cation type, and electrolyte concentration." Environmental Pollution 235 (2018): 350-357.
- Wang, Mei, Congrong Yu, Deshan Tang, Jianjun Chen, and Bin Gao. "Effects of Surfactant and Electrolyte Concentrations, Cation Valence, and Temperature on Graphene Oxide Retention and Transport in Saturated Porous Media." Water, Air, & Soil Pollution 230, no. 1 (2019): 21.
- Chumakova, Natalia A., Anastasya T. Rebrikova, Alexandr V. Talyzin, Nikita A. Paramonov, Andrey Kh Vorobiev, and Mikhail V. Korobov. "Properties of Graphite Oxide Powders and Membranes as Revealed by Electron Paramagnetic Resonance Spectroscopy." The Journal of Physical Chemistry C 122, no. 39 (2018): 22750-22759.
- Wu, Zheng-Yuan, Shiang-Feng Tang, Hong-Yuan Zeng, Wen-Jen Lin, Tzu-Chiang Chen, and Yau-Tang Gau. "Infrared response of vanadium oxide (VOx)/SiNx/reduced graphene oxide (rGO) composite microbolometer." Microelectronics Reliability 91 (2018): 313-318.
- Dong, Shunan, Bin Gao, Yuanyuan Sun, Hongyan Guo, Jianfeng Wu, Shaohua Cao, and Jichun Wu. "Visualization of graphene oxide transport in two-dimensional homogeneous and heterogeneous porous media." Journal of hazardous materials 369 (2019): 334-341.
- Zhang, Zijie, and Juewen Liu. "An engineered one-site aptamer with higher sensitivity for label-free detection of adenosine on graphene oxide." Canadian Journal of Chemistry 96, no. 11 (2018): 957-963.
- Seekaew, Yotsarayuth, Weeraphat Pon-On, and Chatchawal Wongchoosuk. "Ultrahigh Selective Room-Temperature Ammonia Gas Sensor Based on Tin–Titanium Dioxide/reduced Graphene/Carbon Nanotube Nanocomposites by the Solvothermal Method." ACS omega 4, no. 16 (2019): 16916-16924.
- Berning, T.; Bessarabov, D. GOMEA: A Conceptual Design of a Membrane Electrode Assembly for a Proton Exchange Membrane Electrolyzer. Membranes 2023, 13, 614. https://doi.org/10.3390/membranes13070614
|
-
Research Citations of ACS Material Products
- Dedelaite, Lina, et al. “Surfaces functionalized by graphene oxide nanosheets for single cell investigations.” Sensors and Actuators B: Chemical, vol. 255, 2018, pp. 1735–1743., doi:10.1016/j.snb.2017.08.187.
- Trinidad, Josh, et al. “Solvent presence and its impact on the lap-Shear strength of SDS-Decorated graphene hybrid electrically conductive adhesives.” International Journal of Adhesion and Adhesives, vol. 78, 2017, pp. 102–110., doi:10.1016/j.ijadhadh.2017.06.012.
- Kahyaoglu, Leyla Nesrin, and Jenna L. Rickus. "Robust Covalent Coupling Scheme for the Development of FRET Aptasensor based on Amino-Silane-Modified Graphene Oxide." Langmuir 34, no. 48 (2018): 14586-14596.
- Lazarević-Pašti, Tamara, Vladan Anićijević, Miloš Baljozović, Dragana Vasić Anićijević, Sanjin Gutić, Vesna Vasić, Natalia V. Skorodumova, and Igor A. Pašti. "The impact of the structure of graphene-based materials on the removal of organophosphorus pesticides from water." Environmental Science: Nano (2018).
- Chumakova, Natalia A., Anastasya T. Rebrikova, Alexandr V. Talyzin, Nikita A. Paramonov, Andrey Kh Vorobiev, and Mikhail V. Korobov. "Properties of Graphite Oxide Powders and Membranes as Revealed by Electron Paramagnetic Resonance Spectroscopy." The Journal of Physical Chemistry C 122, no. 39 (2018): 22750-22759.
- Gao, Xianfeng, Fenfen Wang, Sam Gollon, and Chis Yuan. "Micro Silicon-Graphene-CNT anode for full cell lithium ion battery." (2018).
- Berning, T.; Bessarabov, D. GOMEA: A Conceptual Design of a Membrane Electrode Assembly for a Proton Exchange Membrane Electrolyzer. Membranes 2023, 13, 614. https://doi.org/10.3390/membranes13070614
|
-
FAQ
1. What is the best method to dissolve/etch an AAO Template?
There are two methods that we recommend. Please make the best adjustments based on your own experiment.
Slow Removal: Depending on the microstructure evolution, a slow removal of AAO layer may be preferred. This can be achieved by using 5 wt% phosphate acid at 30º Celsius. Usually, this process is suitable for Single-Pass AAO.
Rapid removal: Use heated 3M NaOH or HCl solution, depending on your specific needs.
2. What's the thickness of the Al substrate?
The Al substrate is 150microns thick.
3. What is the best method for cutting an AAO Template?
The method we recommend involves using a metal ruler, a sharp knife or blade, a clean cloth (specialized cleanroom wipes suggested), and a hard flat surface (glass or steel).
Cutting Method:
1. Cover the hard surface with a cleanroom wipe or clean cloth.
2. Place the AAO sample on the wipe with the Al substrate (back side) facing up.
3. Align the metal ruler parallel to the cut you want to make.
4. Press down firmly on the ruler and slice the AAO sample several times along the edge of the ruler.
5. If the AAO sample is not cut through completely, slowly and carefully bend the template until it comes apart.
6. Finally, the AAO sample can be cleaned by ultrasonic cleaning <30 seconds using acetone, ethanol, isopropyl alcohol or deionized water.
Research Citations of ACS Material Products
- Jaworski, Nazariy, and Nazariy Andrushchak. “A method of nanoporous anodic aluminum oxide structure modeling based on Bezier curves generation.” 2017 14th International Conference The Experience of Designing and Application of CAD Systems in Microelectronics (CADSM), 2017, doi:10.1109/cadsm.2017.7916085.
- Jaworski, Nazariy, Marek Iwaniec, and Mykhaylo Lobur. "Implementation Features of Fibrous Composite Microlevel Structure Model Construction Based on Bezier Curves Generation and OpenCL Technology Usage." Machine Dynamics Research 41 (2017).
- Fleming, Evan, Feng Du, Eric Ou, Liming Dai, and Li Shi. "Thermal conductivity of carbon nanotubes grown by catalyst-free chemical vapor deposition in nanopores." Carbon 145 (2019): 195-200.
- Fleming, Evan. "Multi-scale investigation of composite materials for enhanced phase change thermal storage systems." PhD diss., 2018.
- Liu, Cong. "Nanoporous materials chemistry: From gas separation to high pressure chemistry." (2017).
|
-
FAQs
1. Is the SSZ-13 a chabasite zeolite?
Yes, it is CHA type zeolite.
2. For SSZ-13 (NH4+ Form), does any NH4+ remain after synthesis?
No residual NH4 + remains after synthesis.
3. What is the difference between H+ form and NH4+ form of SSZ-13?
The NH4+ form of SSZ-13 is baked at a high temperature to obtain the H+ form of SSZ-13.
4. What is the tap density for types HB and HC of SSZ-13?
The tap density for type HB is 0.453g/cm3, and the tap density for type HC is 0.627g/cm3.
|
-
FAQ
1.What are the synthesis procedures of Ti3C2Tx MXene?
The preparation procedure is to first etch the Ti3AlC2 powder raw with hydrofluoric acid. Then it is freeze-dried to obtain the powder.
|
-
Research Citations of ACS Material Products
- Ghasemi-Kahrizsangi, Salman, et al. “Impact of Titania nanoparticles addition on the microstructure and properties of MgO-C refractories.” Ceramics International, vol. 43, no. 17, 2017, pp. 15472–15477., doi:10.1016/j.ceramint.2017.08.094.
- Farain, Kasra, Ali Esfandiar, and Alireza Z. Moshfegh. "Universal rotation of nanowires in static uniform electric fields in viscous dielectric liquids." Applied Physics Letters 113, no. 6 (2018): 063101.
- Tybrandt, Klas, Dion Khodagholy, Bernd Dielacher, Flurin Stauffer, Aline F. Renz, György Buzsáki, and János Vörös. "High‐density stretchable electrode grids for chronic neural recording." Advanced Materials 30, no. 15 (2018): 1706520.
- Stauffer, Flurin, Qiang Zhang, Klas Tybrandt, Byron Llerena Zambrano, Julian Hengsteler, André Stoll, Camill Trüeb et al. "Soft Electronic Strain Sensor with Chipless Wireless Readout: Toward Real‐Time Monitoring of Bladder Volume." Advanced Materials Technologies 3, no. 6 (2018): 1800031.
|
-
FAQ
1. What is the source of H+ in the Type A of TS-1?
H+ comes from an organic amine contained in the raw material.
2. Is the pH of TS-1 neutral?
Yes, TS-1 is neutral.
3. Is the Powder (Type A) form of the Titanium Silicate-1 neutral or is it acidic?
The Titanium Silacate-1 powder (Type A) form is weakly acidic.
Research Citations of ACS Material Products
- Mukherjee, Mitrajit. Process of Making Olefins or Alkylate by Reaction of Methanol and/or DME or by Reaction of Methanol and/or DME and Butane.
- Rodenas, Y., R. Mariscal, J. L. G. Fierro, D. Martín Alonso, J. A. Dumesic, and M. López Granados. "Improving the production of maleic acid from biomass: TS-1 catalysed aqueous phase oxidation of furfural in the presence of γ-valerolactone." Green Chemistry 20, no. 12 (2018): 2845-2856.
- Rodenas, Y., J. L. G. Fierro, R. Mariscal, M. Retuerto, and M. López Granados. "Post-synthesis Treatment of TS-1 with TPAOH: Effect of Hydrophobicity on the Liquid-Phase Oxidation of Furfural to Maleic Acid." Topics in Catalysis (2019): 1-10.
- Bregante, Daniel T., Alayna M. Johnson, Ami Y. Patel, E. Zeynep Ayla, Michael J. Cordon, Brandon C. Bukowski, Jeffrey Greeley, Rajamani Gounder, and David W. Flaherty. "Cooperative Effects between Hydrophilic Pores and Solvents: Catalytic Consequences of Hydrogen Bonding on Alkene Epoxidation in Zeolites." Journal of the American Chemical Society (2019).
|
-
FAQ
1. What is the thickness of PMMA?
About 500nm.
2. How do I cut the Trivial Transfer® Graphene into small pieces?
Step 1. Release the TTG into DI water and pick it up on filter paper. Step 2. Keep the filter paper wet‚ cut the TTG into the desired sizes with scissors. Step 3. Return the pieces to the water and they should release once again for transfer to another substrate.
3. What's the green substrate and wet cloth?
The green substrate is a pure PET substrate to help remove bubbles/wrinkles if there is any on the TTG if it causes problems during the transfer. Please contact us for the demo video if necessary. If not, you can just ignore it and it is included in the package just in case.
The wet cloth in the package is to keep TTG in a good quality during storage and transportation.
4. What if the TTG is folded or rolled up?
Please contact us for the demo video if necessary.
Research Citations of ACS Material Products
- Chen, Ruiyi, et al. “Co-Percolating Graphene-Wrapped Silver Nanowire Network for High Performance, Highly Stable, Transparent Conducting Electrodes.” Advanced Functional Materials, vol. 23, no. 41, 2013, pp. 5150–5158., doi:10.1002/adfm.201300124.
- O’Hern, Sean C., et al. “Selective Molecular Transport through Intrinsic Defects in a Single Layer of CVD Graphene.” ACS Nano, vol. 6, no. 11, Sept. 2012, pp. 10130–10138., doi:10.1021/nn303869m.
- Yoo, Jae-Hyuck, et al. “Graphene folds by femtosecond laser ablation.” Applied Physics Letters, vol. 100, no. 23, Apr. 2012, p. 233124., doi:10.1063/1.4724213.
- Longchamp, Jean-Nicolas, et al. “Low-Energy electron transmission imaging of clusters on free-Standing graphene.” Applied Physics Letters, vol. 101, no. 11, Oct. 2012, p. 113117., doi:10.1063/1.4752717.
- Chen, Xu-Dong, et al. “High-Quality and efficient transfer of large-Area graphene films onto different substrates.” Carbon, vol. 56, May 2013, pp. 271–278., doi:10.1016/j.carbon.2013.01.011.
- Ye, Qing., et al. “Polarization-Dependent optical absorption of graphene under total internal reflection.” Applied Physics Letters, vol. 102, no. 2, doi:10.1063/1.4776694.
- Longchamp, Jean-Nicolas, et al. “Ultraclean freestanding graphene by platinum-Metal catalysis.” Journal of Vacuum Science & Technology B, Nanotechnology and Microelectronics: Materials, Processing, Measurement, and Phenomena, vol. 31, no. 2, 2013, p. 020605., doi:10.1116/1.4793746.
- Wang, Yung Yu, and Peter J. Burke. “A large-Area and contamination-Free graphene transistor for liquid-Gated sensing applications.” Applied Physics Letters, vol. 103, no. 5, 2013, p. 052103., doi:10.1063/1.4816764.
- Wang, Peng, et al. “Accurate layers determination of graphene on transparent substrate based on polarization-Sensitive absorption effect.” Applied Physics Letters, vol. 103, no. 18, 2013, p. 181902., doi:10.1063/1.4827812.
- Zhou, Rong, et al. “Large-Energy, narrow-Bandwidth laser pulse at 1645 nm in a diode-Pumped Er:YAG solid-State laser passively Q-Switched by a monolayer graphene saturable absorber.” Applied Optics, vol. 53, no. 2, Sept. 2014, p. 254., doi:10.1364/ao.53.000254.
- Choi, Duyoung, et al. “Nanopatterned Graphene Field Effect Transistor Fabricated Using Block Co-Polymer Lithography.” Materials Research Letters, vol. 2, no. 3, Sept. 2014, pp. 131–139., doi:10.1080/21663831.2013.876676.
- Srisonphan, Siwapon, et al. “Space charge neutralization by electron-Transparent suspended graphene.” Scientific Reports, vol. 4, no. 1, 2014, doi:10.1038/srep03764.
- Joiner, C. A., et al. “Cleaning graphene with a titanium sacrificial layer.” Applied Physics Letters, vol. 104, no. 22, Feb. 2014, p. 223109., doi:10.1063/1.4881886.
- Roy, T., et al. “Tunneling characteristics in chemical vapor deposited graphene–hexagonal boron nitride–graphene junctions.” Applied Physics Letters, vol. 104, no. 12, 2014, p. 123506., doi:10.1063/1.4870073.
- Burke, Peter J. “Charging the Quantum Capacitance of Graphene with a Single Biological Ion Channel.” Biophysical Journal, vol. 106, no. 2, 2014, doi:10.1016/j.bpj.2013.11.2796.
- Li, Peining, et al. “Graphene-Enhanced Infrared Near-Field Microscopy.” Nano Letters, vol. 14, no. 8, 2014, pp. 4400–4405., doi:10.1021/nl501376a.
- Boutilier, Michael S. H., et al. “Implications of Permeation through Intrinsic Defects in Graphene on the Design of Defect-Tolerant Membranes for Gas Separation.” ACS Nano, vol. 8, no. 1, Mar. 2014, pp. 841–849., doi:10.1021/nn405537u.
- Lee, Jiye, et al. “Switching Individual Quantum Dot Emission through Electrically Controlling Resonant Energy Transfer to Graphene.” Nano Letters, vol. 14, no. 12, 2014, pp. 7115–7119., doi:10.1021/nl503587z.
- Srisonphan, Siwapon, and Komsan Hongesombut. “Tuning the ballistic electron transport of spatial graphene–metal sandwich electrode on a vacuum-Silicon-Based device.” RSC Advances, vol. 5, no. 3, 2015, pp. 2032–2037., doi:10.1039/c4ra09503k.
- Hui, Fei, et al. “Mechanical properties of locally oxidized graphene electrodes.” Archive of Applied Mechanics, vol. 85, no. 3, 2014, pp. 339–345., doi:10.1007/s00419-014-0957-4.
- Fujimoto, A, et al. “Negative magnetoresistance in Ti-Cleaned single-Layer graphene.” Journal of Physics: Conference Series, vol. 603, 2015, p. 012021., doi:10.1088/1742-6596/603/1/012021.
- Thareja, Vrinda, et al. “Electrically Tunable Coherent Optical Absorption in Graphene with Ion Gel.” Nano Letters, vol. 15, no. 3, 2015, pp. 1570–1576., doi:10.1021/nl503431d.
- Ye, X. H., et al. “Corrosion resistance of graphene directly and locally grown on bulk nickel substrate by laser irradiation.” RSC Advances, vol. 5, no. 45, 2015, pp. 35384–35390., doi:10.1039/c5ra01267h.
- Horvath, Cameron . “Fabrication and Characterization of Edge-Conformed Graphene-Silicon Waveguides.” IEEE Photonics Technology Letters, vol. 27, no. 6, 6 Jan. 2015, pp. 585–587., doi:10.1109/LPT.2014.2385757.
- Ye, Qing. “Polarization-Dependent optical absorption of graphene under total internal reflection.” Applied Physics Letters, vol. 102, no. 2, Jan. 2013, doi:10.1063/1.4776694.
- Zheng, Guanpeng, et al. “Improved Transfer Quality of CVD-Grown Graphene by Ultrasonic Processing of Target Substrates: Applications for Ultra-Fast Laser Photonics.” ACS Applied Materials & Interfaces, vol. 5, no. 20, Sept. 2013, pp. 10288–10293., doi:10.1021/am403205v.
- Zeng, Yong, et al. “Investigate the interface structure and growth mechanism of high quality ZnO films grown on multilayer graphene layers.” Applied Surface Science, vol. 301, 2014, pp. 391–395., doi:10.1016/j.apsusc.2014.02.088.
- Tang, Pinghua, et al. “Stable high-Energy Q-Switched resonantly diode-Pumped Er:YAG laser at 1645 nm.” OSA Publishing, vol. 53, no. 32, 2014, pp. 7773–7777., doi:10.1364/AO.53.007773.
- Watanabe, Hiroshi, et al. “Layer number dependence of carrier lifetime in graphenes observed using time-Resolved mid-Infrared luminescence.” Chemical Physics Letters, vol. 637, 2015, pp. 58–62., doi:10.1016/j.cplett.2015.07.046.
- He, Yingbo, et al. “Strongly enhanced Raman scattering of graphene by a single gold nanorod.” Applied Physics Letters, vol. 107, no. 5, Mar. 2015, p. 053104., doi:10.1063/1.4927759.
- Choi, Duyoung, et al. “Uniformly Nanopatterned Graphene Field-Effect Transistors with Enhanced Properties.” Nanoscale Research Letters, vol. 10, no. 1, Nov. 2015, doi:10.1186/s11671-015-0976-2.
- Miao, Lili, et al. “Broadband ultrafast nonlinear optical response of few-Layers graphene: toward the mid-Infrared regime.” Photonics Research, vol. 3, no. 5, 2015, p. 214., doi:10.1364/prj.3.000214.
- Hu, Jianchen, et al. “Improvement of the electrical contact resistance at rough interfaces using two dimensional materials.” Journal of Applied Physics, vol. 118, no. 21, July 2015, p. 215301., doi:10.1063/1.4936366.
- Jain, Tarun, et al. “Heterogeneous sub-Continuum ionic transport in statistically isolated graphene nanopores.” Nature Nanotechnology, vol. 10, no. 12, May 2015, pp. 1053–1057., doi:10.1038/nnano.2015.222.
- Srisonphan, Siwapon, and Komsan Hongesombut. “Tuning the ballistic electron transport of spatial graphene–metal sandwich electrode on a vacuum-Silicon-Based device.” RSC Advances, vol. 5, no. 3, 2015, pp. 2032–2037., doi:10.1039/c4ra09503k.
- Liu, Xiangjiang, et al. “Compact Shielding of Graphene Monolayer Leads to Extraordinary SERS-Active Substrate with Large-Area Uniformity and Long-Term Stability.” Scientific Reports, vol. 5, no. 1, 2015, doi:10.1038/srep17167.
- Hui, Fei, et al. “Moving graphene devices from lab to market: advanced graphene-Coated nanoprobes.” Nanoscale, vol. 8, no. 16, 2016, pp. 8466–8473., doi:10.1039/c5nr06235g.
- Ye, Xiaohui, et al. “Protecting carbon steel from corrosion by laser in situ grown graphene films.” Carbon, vol. 94, 2015, pp. 326–334., doi:10.1016/j.carbon.2015.06.080.
- Wu, Man, et al. “Wavelength switchable graphene Q-Switched fiber laser with cascaded fiber Bragg gratings.” Optics Communications, vol. 368, 2016, pp. 81–85., doi:10.1016/j.optcom.2016.01.069.
- Mackin, Charles, and Tomás Palacios. “Large-Scale sensor systems based on graphene electrolyte-Gated field-Effect transistors.” The Analyst, vol. 141, no. 9, 2016, pp. 2704–2711., doi:10.1039/c5an02328a.
- Politou, Maria, et al. “Multi-Layer graphene interconnect.” IEEE Xplore Digital Library , 11 July 2016, doi:10.1109/IITC-AMC.2016.7507731.
- Cao, Zhengmin, et al. “Nano-Gap between a gold tip and nanorod for polarization dependent surface enhanced Raman scattering.” Applied Physics Letters, vol. 109, no. 23, May 2016, p. 233103., doi:10.1063/1.4971832.
- Deng, Xiangquan, et al. “Terahertz-Induced photothermoelectric response in graphene-Metal contact structures.” Journal of Physics D: Applied Physics, vol. 49, no. 42, 2016, p. 425101., doi:10.1088/0022-3727/49/42/425101.
- Guo, Chu‐Cai, et al. “Experimental Demonstration of Total Absorption over 99% in the Near Infrared for Monolayer‐Graphene‐Based Subwavelength Structures.” Advanced Optical Materials, 1 Sept. 2016, onlinelibrary.wiley.com/doi/10.1002/adom.201600481/full.
- Hu, Jianbo, et al. “Rippling ultrafast dynamics of suspended 2D monolayers, graphene.” Proceedings of the National Academy of Sciences, vol. 113, no. 43, Oct. 2016, doi:10.1073/pnas.1613818113.
- Li, Cheng, et al. “Measurement of the Adhesion Energy of Pressurized Graphene Diaphragm Using Optical Fiber Fabry–Perot Interference.” IEEE Sensors Journal, vol. 16, no. 10, 2016, pp. 3664–3669., doi:10.1109/jsen.2016.2536783.
- Yuan, Jun, et al. “Modulation of far-Infrared light transmission by graphene-Silicon Schottky junction.” Optical Materials Express, vol. 6, no. 12, 2016, p. 3908., doi:10.1364/ome.6.003908.
- Li, Cheng, et al. “Measurement of thermal expansion coefficient of graphene diaphragm using optical fiber Fabry–Perot interference.” Measurement Science and Technology, vol. 27, no. 7, 2016, p. 075102., doi:10.1088/0957-0233/27/7/075102.
- Kafiah, Feras M., et al. “Monolayer graphene transfer onto polypropylene and polyvinylidenedifluoride microfiltration membranes for water desalination.” Desalination, vol. 388, 2016, pp. 29–37., doi:10.1016/j.desal.2016.02.027.
- Wu, Xiangyu. “Doping of graphene for the application in nano-Interconnect.” Microelectronic Engineering, vol. 167, 5 Jan. 2017, pp. 42–46., doi:10.1016/j.mee.2016.10.013.
- Liu, Siyang, et al. “Atomic emission spectroscopy of electrically triggered exploding nanoparticle analytes on graphene/SiO2/Si substrate.” Journal of Vacuum Science & Technology B, Nanotechnology and Microelectronics: Materials, Processing, Measurement, and Phenomena, vol. 34, no. 6, doi:10.1116/1.4964819.
- Sattler, Klaus D. Carbon nanomaterials sourcebook. Vol. 1, CRC Press, 2016.
- Politou, Maria. “Evaluation of multilayer graphene for advanced interconnects.” Microelectronic Engineering, vol. 167, 5 Jan. 2017, pp. 1–5., doi:10.1016/j.mee.2016.09.011.
- Hwang, Michael T., et al. “Highly specific SNP detection using 2D graphene electronics and DNA strand displacement.” Proceedings of the National Academy of Sciences, vol. 113, no. 26, 2016, pp. 7088–7093., doi:10.1073/pnas.1603753113.
- Nieto, Andy, et al. “Graphene reinforced metal and ceramic matrix composites: a review.” International Materials Reviews, vol. 62, no. 5, 2017, pp. 241–302., doi:10.1080/09506608.2016.1219481.
- Khorasaninejad, M., et al. “Highly Enhanced Raman Scattering of Graphene using Plasmonic Nano-Structure.” Scientific Reports, vol. 3, no. 1, 2013, doi:10.1038/srep02936.
- Kunz, Daniel A., et al. “Space-Resolved In-Plane Moduli of Graphene Oxide and Chemically Derived Graphene Applying a Simple Wrinkling Procedure (Adv. Mater. 9/2013).” Advanced Materials, vol. 25, no. 9, Apr. 2013, pp. 1336–1336., doi:10.1002/adma.201370060.
- Peng, Shuhua, et al. “Microwetting of Supported Graphene on Hydrophobic Surfaces Revealed by Polymerized Interfacial Femtodroplets.” Langmuir, vol. 30, no. 33, 2014, pp. 10043–10049., doi:10.1021/la5022774.
- Luo, Wen, et al. “Single-Layer Graphene as an Effective Mediator of the Metal–Support Interaction.” The Journal of Physical Chemistry Letters, vol. 5, no. 11, 2014, pp. 1837–1844., doi:10.1021/jz500425j.
- Suemoto, Tohru, et al. “Thickness dependent hot-Phonon effects observed by femtosecond mid-Infrared luminescence in graphene.” 19th International Conference on Ultrafast Phenomena, 2014, doi:10.1364/up.2014.07.mon.p1.32.
- Zhou, Haosen, et al. "Influence of CO2 On the Stability of Discharge Performance for Li-Air Battery with Hybrid Electrolyte Based On the Graphene Sheet”, Conference: 224th ECS Meeting. Oct. 2013, doi:10.1039/c3ra47258b.
- Thareja, Vrinda. “Electrically Tunable Optical Absorption in a Graphene-Based Salisbury Screen.” CORNELL UNIVERSITY LIBRARY, 7 Sept. 2014.
- Horvath, Cameron S. “Theses and Dissertations: Light Propagation and Photothermal Nonlinearity in Graphene-Si Waveguides.” University of Alberta Libraries, 26 Sept. 2013, doi:10.7939/R3ST1N .
- Li, Cheng, et al. “Interference characteristics in a Fabry–Perot cavity with graphene membrane for optical fiber pressure sensors.” Microsystem Technologies, vol. 21, no. 11, 2014, pp. 2297–2306., doi:10.1007/s00542-014-2333-2.
- Kou, Jun-Long, et al. “Platform for enhanced light–graphene interaction length and miniaturizing fiber stereo devices.” Optica, vol. 1, no. 5, 2014, p. 307., doi:10.1364/optica.1.000307.
- Karnik, Rohit. “Ionic and Molecular Transport Through Graphene Membranes.” Transport and Reactivity of Solutions in Confined Hydrosystems NATO Science for Peace and Security Series C: Environmental Security, Dec. 2013, pp. 95–102., doi:10.1007/978-94-007-7534-3_8.
- Warren, A.J, et al. “In vacuo X-Ray data collection from graphene-Wrapped protein crystals.” Acta Crystallographica Section D STRUCTURAL BIOLOGY, vol. 71, 1 Oct. 2015, pp. 2079–2088., doi:10.1107/S1399004715014194.
- Wen, Chenyu, et al. “Assessing kinetics of surface adsorption–desorption of gas molecules via electrical measurements.” Sensors and Actuators B: Chemical, vol. 223, 2016, pp. 791–798., doi:10.1016/j.snb.2015.10.019.
- Li, Cheng, et al. “Analyzing the applicability of miniature ultra-High sensitivity Fabry–Perot acoustic sensor using a nanothick graphene diaphragm.” Measurement Science and Technology, vol. 26, no. 8, Oct. 2015, p. 085101., doi:10.1088/0957-0233/26/8/085101.
- Li, Cheng, et al. “Analyzing the temperature sensitivity of Fabry-Perot sensor using multilayer graphene diaphragm.” OSA Publishing/ Optics Express, vol. 23, no. 21, 2015, pp. 27494–27502 ., doi:10.1364/OE.23.027494.
- Lau, K. Y., et al. “High Signal-To-Noise Ratio Q-Switching Erbium Doped Fiber Laser Pulse Emission Utilizing Single Layer Trivial Transfer Graphene Film Saturable Absorber.” Jurnal Teknologi, vol. 78, no. 3, 2016, doi:10.11113/jt.v78.7478.
- Zheng, Shijun, et al. “Acoustic charge transport induced by the surface acoustic wave in chemical doped graphene.” Applied Physics Letters, vol. 109, no. 18, 2016, p. 183110., doi:10.1063/1.4967192.
- Deng, Xiangquan, et al. “Terahertz-Induced photothermoelectric response in graphene-Metal contact structures.” Journal of Physics D: Applied Physics, vol. 49, no. 42, 2016, p. 425101., doi:10.1088/0022-3727/49/42/425101.
- Luo, Wen. “Graphene-Coated ZnO and SiO2 as Supports for CoO Nanoparticles with Enhanced Reducibility.” Chemphys, vol. 17, no. 19, 5 Oct. 2016, pp. 3055–3061., doi:10.1002/cphc.201600499.
- Lau, K.y., et al. “Passively mode-Locked soliton femtosecond pulses employing graphene saturable absorber.” Optics & Laser Technology, vol. 94, 2017, pp. 221–227., doi:10.1016/j.optlastec.2017.03.035.
- Fitri, Meika Aidil, et al. “Fabrication of TiO 2 -Graphene photocatalyst by direct chemical vapor deposition and its anti-Fouling property.” Materials Chemistry and Physics, vol. 198, 2017, pp. 42–48., doi:10.1016/j.matchemphys.2017.05.053.
- Li, Cheng, et al. “Nondestructive andin situdetermination of graphene layers using optical fiber Fabry–Perot interference.” Measurement Science and Technology, vol. 28, no. 2, Dec. 2017, p. 025206., doi:10.1088/1361-6501/aa54f8.
- Gao, Xiangyang, et al. “Measuring Graphene Adhesion on Silicon Substrate by Single and Dual Nanoparticle-Loaded Blister.” Advanced Materials Interfaces, vol. 4, no. 9, 2017, p. 1601023., doi:10.1002/admi.201601023.
- Tang, Xiaoduan, et al. “Five Orders of Magnitude Reduction in Energy Coupling across Corrugated Graphene/Substrate Interfaces.” ACS Applied Materials & Interfaces, vol. 6, no. 4, July 2014, pp. 2809–2818., doi:10.1021/am405388a.
- Morrow, W. K., et al. “(Invited) The Use of Graphene as a Solid State Diffusion Barrier.” ECS Transactions, vol. 61, no. 4, 2014, pp. 371–379., doi:10.1149/06104.0371ecst.
- Zheng, Haisheng, et al. “Ultrafine Pt nanoparticle induced doping/Strain of single layer graphene: experimental corroboration between conduction and Raman characteristics.” Journal of Materials Science: Materials in Electronics, vol. 26, no. 7, 2015, pp. 4746–4753., doi:10.1007/s10854-015-3043-y.
- Blinco , James P. “Spin-Coated carbon.” Chemical Science, no. 9, 12 June 2013, pp. 3411–3415., doi:10.1039/C3SC51396C.
- Zheng, H., et al. “Effect of Sub 1-Nm Pt Nanoparticle on the Conduction Properties of Graphene Based Field Effect Transistor.” ECS Transactions, vol. 61, no. 39, Jan. 2014, pp. 1–11., doi:10.1149/06139.0001ecst.
- Mckitterick, Christopher B., et al. “Electron-Phonon cooling in large monolayer graphene devices.” Physical Review B, vol. 93, no. 7, Apr. 2016, doi:10.1103/physrevb.93.075410
- Chan, Chun Yu. “Graphene based electrical biosensors for the detection of biomolecules.” The Hong Kong Polytechnic University, 2016.
- Pápa, Z., et al. “Spectroscopic ellipsometric investigation of graphene and thin carbon films from the point of view of depolarization effects.” Applied Surface Science, vol. 421, 2017, pp. 714–721., doi:10.1016/j.apsusc.2016.11.231.
- Hussain, Mushtaque, et al. “The improved piezoelectric properties of ZnO nanorods with oxygen plasma treatment on the single layer graphene coated polymer substrate.” Physica status solidi (a), vol. 211, no. 2, Oct. 2014, pp. 455–459., doi:10.1002/pssa.201300330.
- Kafiah, Feras, et al. “Synthesis of Graphene Based Membranes: Effect of Substrate Surface Properties on Monolayer Graphene Transfer.” Materials, vol. 10, no. 1, 2017, p. 86., doi:10.3390/ma10010086.
- Liang, Ji, et al. “Modulation of acousto-Electric current using a hybrid on-Chip AlN SAW/GFET device.” Applied Physics Letters, vol. 110, no. 24, Dec. 2017, p. 243504., doi:10.1063/1.4986481.
- Zhang, Yingjie, et al. "Electron transport in strain superlattices of graphene." Cornell University Library. 16 Mar. 2017.
- Yan, Xiao-Qing, et al. “Polarization dependence of graphene transient optical response: interplay between incident direction and anisotropic distribution of nonequilibrium carriers.” Journal of the Optical Society of America B, vol. 34, no. 1, 2016, p. 218., doi:10.1364/josab.34.000218.
- Lau, K.Y., et al. “Passively mode-Locked soliton femtosecond pulses employing graphene saturable absorber.” Optics & Laser Technology, vol. 94, 2017, pp. 221–227., doi:10.1016/j.optlastec.2017.03.035.
- Peng, Shuhua, et al. “Microwetting of Supported Graphene on Hydrophobic Surfaces Revealed by Polymerized Interfacial Femtodroplets.” Langmuir, vol. 30, no. 33, 2014, pp. 10043–10049., doi:10.1021/la5022774.
- Horvath, Cameron, et al. “Edge-Conformed silicon-Graphene waveguides: Fabrication and measurements.” 11th International Conference on Group IV Photonics (GFP), 2014, doi:10.1109/group4.2014.6961953.
- Watanabe, Hiroshi, et al. “Femtosecond midi-Infrared luminescence with hot-Phonon effects in graphenes and graphite.”
- Hassan, Saad. Transparent conductive article.
- Liang, Ji, et al. “Manipulation of carriers in graphene using an on-Chip acoustic wave device.” 2017 IEEE International Ultrasonics Symposium (IUS), 2017, doi:10.1109/ultsym.2017.8092898.
- Luo, Wen, et al. “Interaction of bimetallic PtCo layers with bare and graphene-Covered ZnO(0001) supports.” Surface Science, vol. 669, 2018, pp. 64–70., doi:10.1016/j.susc.2017.11.001.
- Jiang, Wen-Shuai, et al. “Preparation of high-Quality graphene using triggered microwave reduction under an air atmosphere.” Journal of Materials Chemistry C, 2018, doi:10.1039/c7tc03957c.
- Dong, Nannan, et al. “Pressure and Temperature Sensor Based on Graphene Diaphragm and Fiber Bragg Gratings.” IEEE Photonics Technology Letters, 2017, pp. 1–1., doi:10.1109/lpt.2017.2786292.
- Huczko, Andrzej, et al. “Efficient one-Pot combustion synthesis of few-Layered graphene.” Physica status solidi (b), vol. 252, no. 11, 2015, pp. 2412–2417., doi:10.1002/pssb.201552233.
- Lu, Bingyu, et al. “Roughened cylindrical gold layer with curve graphene coating for enhanced sensitivity of fiber SPR sensor.” 2017 19th International Conference on Solid-State Sensors, Actuators and Microsystems (TRANSDUCERS), 2017, doi:10.1109/transducers.2017.7994461.
- Fitri, Meika Aidil, et al. “Fabrication of TiO 2 -Graphene photocatalyst by direct chemical vapor deposition and its anti-Fouling property.” Materials Chemistry and Physics, vol. 198, 2017, pp. 42–48., doi:10.1016/j.matchemphys.2017.05.053.
- Bezryadin, A, et al. “Large energy storage efficiency of the dielectric layer of graphene nanocapacitors.” Nanotechnology, vol. 28, no. 49, 2017, p. 495401., doi:10.1088/1361-6528/aa935c.
- Shi, Cheng, et al. “Metamaterial-Based graphene thermal emitter.” Nano Research, June 2017, doi:10.1007/s12274-017-1922-7.
- Mccaffrey, Debra L., et al. “Mechanism of ion adsorption to aqueous interfaces: Graphene/Water vs. air/Water.” Proceedings of the National Academy of Sciences, vol. 114, no. 51, 2017, pp. 13369–13373., doi:10.1073/pnas.1702760114.
- Zhang, Qin, et al. “Negative differential resistance and hysteresis in graphene-Based organic light-Emitting devices.” Journal of Materials Chemistry C, The Royal Society of Chemistry, 1 Jan. 2018, pubs.rsc.org/en/Content/ArticleLanding/2017/TC/C7TC05148D#!
- Miskin, Marc Z., et al. “Graphene-Based bimorphs for micron-Sized, autonomous origami machines.” Proceedings of the National Academy of Sciences, vol. 115, no. 3, Feb. 2018, pp. 466–470., doi:10.1073/pnas.1712889115.
- Bukola, Saheed, et al. “Selective Proton/Deuteron Transport through Nafion|Graphene|Nafion Sandwich Structures at High Current Density.” Journal of the American Chemical Society, vol. 140, no. 5, 2018, pp. 1743–1752., doi:10.1021/jacs.7b10853.
- Brown, Morgan A., et al. “Graphene Biotransistor Interfaced with a Nitrifying Biofilm.” Environmental Science & Technology Letters, vol. 2, no. 4, Mar. 2015, pp. 118–122., doi:10.1021/acs.estlett.5b00025.
- Woo, Sung Oh, and Winfried Teizer. “The effect of electron induced hydrogenation of graphene on its electrical transport properties.” Applied Physics Letters, vol. 103, no. 4, 2013, p. 041603., doi:10.1063/1.4816475.
- Li, Cheng, et al. “Manipulation of Nonlinear Optical Properties of Graphene Bonded Fiber Devices by Thermally Engineering Fermi-Dirac Distribution.” Advanced Optical Materials, vol. 5, no. 21, 2017, doi:10.1002/adom.201770103.
- Chirayath, V. A, et al. “Positron induced electron emission from graphene.” IOP Science, Journal of Physics: Conference Series.
- Lock, Evgeniya H., et al. Stable IR Transparent Conductive Graphene Hybrid Materials and Methods of Making.
- Beechem, Thomas E., et al. “Self-Heating and Failure in Scalable Graphene Devices.” Scientific Reports, vol. 6, no. 1, Sept. 2016, doi:10.1038/srep26457.
- Ma, Yufeng, and Anni Siitonen. Chemical sensor using molecularly-Imprinted single layer graphene.
- Morrow, Wayne K., et al. “Role of graphene interlayers in mitigating degradation of Ni/Au ohmic contact morphology on p-Type GaN.” Vacuum, vol. 128, 2016, pp. 34–38., doi:10.1016/j.vacuum.2016.03.004.
- Nagamanasa, Kandula Hima, et al. “Liquid-Cell Electron Microscopy of Adsorbed Polymers.” Advanced Materials, vol. 29, no. 41, 2017, p. 1703555., doi:10.1002/adma.201703555.
- Hui, Fei. “Variability of graphene devices fabricated using graphene inks: Atomic force microscope tips.” Surface and Coatings Technology, vol. 320, 25 June 2017, doi:10.1016/j.surfcoat.2016.12.020.
- Chirayath, V A, et al. “Investigation of graphene using low energy positron annihilation induced Doppler broadening spectroscopy.” Journal of Physics: Conference Series, vol. 791, 2017, p. 012032., doi:10.1088/1742-6596/791/1/012032.
- Niu, Tianxiao, et al. “Indentation behavior of the stiffest membrane mounted on a very compliant substrate: Graphene on PDMS.” International Journal of Solids and Structures, vol. 132-133, 2018, pp. 1–8., doi:10.1016/j.ijsolstr.2017.05.038.
- Nashed, Ramy, et al. “Ultra-High Mobility in Dielectrically Pinned CVD Graphene.” IEEE Journal of the Electron Devices Society, vol. 4, no. 6, 2016, pp. 466–472., doi:10.1109/jeds.2016.2595498.
- Niu, Tianxiao, et al. “Fracture behavior of graphene mounted on stretchable substrate.” Carbon, vol. 109, 2016, pp. 852–859., doi:10.1016/j.carbon.2016.08.087.
- Boutilier, Michael S H, et al. “Knudsen effusion through polymer-Coated three-Layer porous graphene membranes.” Nanotechnology, vol. 28, no. 18, Oct. 2017, p. 184003., doi:10.1088/1361-6528/aa680f.
- Du, Feng, et al. “Surface stress of graphene layers supported on soft substrate.” Scientific Reports, vol. 6, no. 1, Nov. 2016, doi:10.1038/srep25653.
- Yokaribas, Volkan, et al. “Strain Gauges Based on CVD Graphene Layers and Exfoliated Graphene Nanoplatelets with Enhanced Reproducibility and Scalability for Large Quantities.” Sensors, vol. 17, no. 12, 2017, p. 2937., doi:10.3390/s17122937.
- Kuru, Cihan, et al. “Enhanced Power Conversion Efficiency of Graphene/Silicon Heterojunction Solar Cells Through NiO Induced Doping.” Journal of Nanoscience and Nanotechnology, vol. 16, no. 1, Jan. 2016, pp. 1190–1193., doi:10.1166/jnn.2016.12079.
- Korzeniewski, Carol, Jay P. Kitt, Saheed Bukola, Stephen E. Creager, Shelley D. Minteer, and Joel M. Harris. "Single Layer Graphene for Estimation of Axial Spatial Resolution in Confocal Raman Microscopy Depth Profiling." Analytical chemistry 91, no. 1 (2018): 1049-1055.
- Mackin, Charles E., and Tomás Apostol Palacios. "Sensor systems and related fabrication techniques." U.S. Patent Application 15/773,247, filed November 8, 2018.
- Wu, Ting, Abdullah Alharbi, Takashi Taniguchi, Kenji Watanabe, and Davood Shahrjerdi. "Low-frequency noise in irradiated graphene FETs." Applied Physics Letters 113, no. 19 (2018): 193502.
- Xie, Yadian. "Study on high-purity alumina preparation and its applications." PhD diss., Université du Québec à Chicoutimi, 2017.
- Carmichael, Christopher P., M. Scott Smith, Arthur R. Weeks, and Donald C. Malocha. "Experimental Investigation of Surface Acoustic Wave Acoustoelectric Effect using a Graphene Film on Lithium Niobate." IEEE transactions on ultrasonics, ferroelectrics, and frequency control 65, no. 11 (2018): 2205-2207.
- Laua, K. Y., A. A. Latifb, MH Abu Bakara, and M. A. Mahdia. "HIGH SIGNAL-TO-NOISE RATIO Q-SWITCHING ERBIUM DOPED FIBER LASER PULSE EMISSION UTILIZING SINGLE LAYER TRIVIAL TRANSFER GRAPHENE FILM SATURABLE ABSORBER." JURNAL TEKNOLOGI 78, no. 3 (2016): 129-133.
- Carmichael, Christopher P., M. Scott Smith, Arthur R. Weeks, and Donald C. Malocha. "Experimental Investigation of Surface Acoustic Wave Acoustoelectric Effect using a Graphene Film on Lithium Niobate." IEEE transactions on ultrasonics, ferroelectrics, and frequency control 65, no. 11 (2018): 2205-2207.
- Fujimoto, Akira, Christopher J. Perini, Daiju Terasawa, Akira Fukuda, Yoshiyuki Harada, Shigehiko Sasa, Mitsuaki Yano, and Eric M. Vogel. "Disorder and Weak Localization near Charge Neutral Point in Ti‐cleaned Single‐Layer Graphene." physica status solidi (b) (2019): 1800541.
- Zhao, Jingyi, Zhengmin Cao, Yuqing Cheng, Jianning Xu, Te Wen, Aiqin Hu, Qihuang Gong, and Guowei Lu. "In situ Optical Study of Gold Nanorod Coupling with Graphene." Advanced Optical Materials 6, no. 8 (2018): 1701043.
- Su, Fang, Zhaohao Zhang, Shasha Li, Peian Li, and Tao Deng. "Long-term stability of photodetectors based on graphene field-effect transistors encapsulated with Si3N4 layers." Applied Surface Science 459 (2018): 164-170.
- Ulibarri, Agatha. "Measuring the Double Layer Capacitance of Electrolyte Solutions Using a Graphene Field Effect Transistor." (2018).
- Dong, Nannan, Sumei Wang, Lan Jiang, Yi Jiang, Peng Wang, and Liuchao Zhang. "Pressure and temperature sensor based on graphene diaphragm and fiber Bragg gratings." IEEE Photonics Technology Letters 30, no. 5 (2018): 431-434.
- Lau, K. Y., MH Abu Bakar, AR Md Zain, A. F. Abas, M. T. Alresheedi, and M. A. Mahdi. "Stable multi-wavelength erbium-doped fiber laser assisted by graphene/PMMA thin film." Optics & Laser Technology 105 (2018): 129-134.
- Herekar, Satish. "Systems, methods, and kits to reduce surface heating during tissue treatment." U.S. Patent Application 15/896,886, filed June 28, 2018.
- Xu, Wendao, Lijuan Xie, Jianfei Zhu, Longhua Tang, Ranjan Singh, Chen Wang, Yungui Ma, Hou-Tong Chen, and Yibin Ying. "Terahertz biosensing with a graphene-metamaterial heterostructure platform." Carbon 141 (2019): 247-252.
- Deng, Tao, Zhaohao Zhang, Yaxuan Liu, Yingxin Wang, Fang Su, Shasha Li, Yang Zhang et al. "Three-dimensional graphene field-effect transistors as high-performance photodetectors." Nano letters (2019).
- Barnard, H. R., E. Zossimova, N. H. Mahlmeister, L. M. Lawton, I. J. Luxmoore, and G. R. Nash. "Boron nitride encapsulated graphene infrared emitters." Applied Physics Letters 108, no. 13 (2016): 131110.
- Wu, Xiangyu, Inge Asselberghs, Cedric Huyghebaert, Antonino Contino, Bart Soree, Marc Heyns, and Zsolt Tokei. "Graphene Interconnects-High Performance Twisted 20 nm Graphene Ribbons." In 2018 IEEE International Interconnect Technology Conference (IITC), pp. 25-27. IEEE, 2018.
- Li, Cheng, Xiyu Yu, Tian Lan, Jian Liu, and Shangchun Fan. "Insensitivity to Humidity in Fabry–Perot Sensor With Multilayer Graphene Diaphragm." IEEE Photonics Technology Letters 30, no. 6 (2018): 565-568.
- Dong, Nannan, and Bo Yang. "Simultaneous Measurement of Pressure and Temperature Based on Graphene." In IOP Conference Series: Materials Science and Engineering, vol. 382, no. 5, p. 052011. IOP Publishing, 2018.
- Zhang, Yingjie, Youngseok Kim, Matthew J. Gilbert, and Nadya Mason. "Electronic transport in a two-dimensional superlattice engineered via self-assembled nanostructures." npj 2D Materials and Applications 2, no. 1 (2018): 31.
- Mackin, Charles Edward. "Graphene chemical and biological sensors: modeling, systems, and applications." PhD diss., Massachusetts Institute of Technology, 2018.
- Yin, Yin, Jinbo Pang, Jiawei Wang, Xueyi Lu, Qi Hao, Ehsan Saei Ghareh Naz, Xinxing Zhou, Libo Ma, and Oliver G. Schmidt. "Graphene-Activated Optoplasmonic Nanomembrane Cavities for Photodegradation Detection." ACS applied materials & interfaces (2019).
- Andoy, Nesha M., Marcin S. Filipiak, Daniel Vetter, Óscar Gutiérrez‐Sanz, and Alexey Tarasov. "Graphene‐Based Electronic Immunosensor with Femtomolar Detection Limit in Whole Serum." Advanced Materials Technologies 3, no. 12 (2018): 1800186.
- Schweizer, Peter, Christian Dolle, and Erdmann Spiecker. "In situ manipulation and switching of dislocations in bilayer graphene." Science advances 4, no. 8 (2018): eaat4712.
- Hutzler, Andreas, Christian David Matthus, Christian Dolle, Mathias Rommel, Michael PM Jank, Erdmann Spiecker, and Lothar Frey. "Large-Area Layer Counting of Two-Dimensional Materials Evaluating the Wavelength Shift in Visible-Reflectance Spectroscopy." The Journal of Physical Chemistry C (2019).
- Hu, Ming, Yucong Yan, Kun Huang, Afzal Khan, Xiaodong Qiu, Dikai Xu, Hui Zhang, Xuegong Yu, and Deren Yang. "Performance Improvement of Graphene/Silicon Photodetectors Using High Work Function Metal Nanoparticles with Plasma Effect." Advanced Optical Materials 6, no. 9 (2018): 1701243.
- Hu, Shu-Kai, Fang-Yen Lo, Chih-Chen Hsieh, and Ling Chao. "Sensing Ability and Formation Criterion of Fluid Supported Lipid Bilayer Coated Graphene Field-Effect Transistors." ACS sensors (2019).
- Nemani, Srinivasa Kartik, Dayong Chen, Marwan H. Mohamed, and Hossein Sojoudi. "Stretchable and hydrophobic electrochromic devices using wrinkled graphene and PEDOT: PSS." Journal of Nanomaterials 2018 (2018).
- Wang, Kenan. "The Incorporation of Graphene to Lithium Cobalt Oxide as a Cathode to Improve the Performance of Lithium Ion Batteries." (2018).
- Cho, Hoduk. "The Use of Liquid Phase Transmission Electron Microscopy for Quantifying Interactions Between Colloidal Nanoparticles and Visualizing Their Self-Assembled Structures." PhD diss., UC Berkeley, 2018.
- Goldflam, Michael D., Isaac Ruiz, Stephen W. Howell, Joel R. Wendt, Michael B. Sinclair, David W. Peters, and Thomas E. Beechem. "Tunable dual-band graphene-based infrared reflectance filter." Optics express 26, no. 7 (2018): 8532-8541.
- Hutzler, A., C. D. Matthus, M. Rommel, and L. Frey. "Generalized approach to design multi-layer stacks for enhanced optical detectability of ultrathin layers." Applied Physics Letters 110, no. 2 (2017): 021909.
- Hutzler, Andreas, Tilo Schmutzler, Michael PM Jank, Robert Branscheid, Tobias Unruh, Erdmann Spiecker, and Lothar Frey. "Unravelling the Mechanisms of Gold–Silver Core–Shell Nanostructure Formation by in Situ TEM Using an Advanced Liquid Cell Design." Nano letters 18, no. 11 (2018): 7222-7229.
- Hutzler, Andreas, Birk Fritsch, Michael PM Jank, Robert Branscheid, Erdmann Spiecker, and Martin März. "Preparation of Graphene-Supported Microwell Liquid Cells for In Situ Transmission Electron Microscopy." JoVE (Journal of Visualized Experiments) 149 (2019): e59751.
- Hutzler, Andreas, Birk Fritsch, Michael PM Jank, Robert Branscheid, R. Christian Martens, Erdmann Spiecker, and Martin März. "In Situ Liquid Cell TEM Studies on Etching and Growth Mechanisms of Gold Nanoparticles at a Solid–Liquid–Gas Interface." Advanced Materials Interfaces (2019).
- Lee, I-Kon, Hung-Hua Sheu, and Heng-Yao Hsu. "The effects of graphene content on the mechanical properties and thermal conductivity of Inconel 718 superalloy brazed using BNi-2/graphene composite filler metal." Results in Physics (2019): 102828.
|
-
Q&A
1. What does "dry method" refer to and is there any more information that can be given regarding the preparation method? Unfortunately, no other information can be provided regarding the preparation method. Its a trade secret!
2. Does ACS give a classification of the crystal structure of the powder? It is a hexagonal crystal system.
3. Is any information available about specific surface area and bulk density regarding this material? BET was not tested, bulk density is 0.57g / cm³ and tap density is 1.08g / cm³.
Research Citations of ACS Material Products
- Lu, Chang, et al. “Comparison of MoS2, WS2, and Graphene Oxide for DNA Adsorption and Sensing.” Langmuir, vol. 33, no. 2, May 2017, pp. 630–637., doi:10.1021/acs.langmuir.6b04502.
- Liu, Yibo, and Juewen Liu. “Hybrid nanomaterials of WS2 or MoS2 nanosheets with liposomes: biointerfaces and multiplexed drug delivery.” Nanoscale, vol. 9, no. 35, 2017, pp. 13187–13194.
- Wang, Liu, et al. “Transition Metal Dichalcogenide Nanosheets for Visual Monitoring PCR Rivaling a Real-Time PCR Instrument.” ACS Applied Materials & Interfaces, vol. 10, no. 5, 2018, pp. 4409–4418., doi:10.1021/acsami.7b15746.
- Giust, Davide, María Isabel Lucío, Otto L. Muskens, and Antonios G. Kanaras. "Interactions of DNA coated upconversion nanoparticles with 2D materials." In Colloidal Nanoparticles for Biomedical Applications XIII, vol. 10507, p. 105070U. International Society for Optics and Photonics, 2018.
- Lau, K. Y., A. A. Latif, MH Abu Bakar, F. D. Muhammad, M. F. Omar, and M. A. Mahdi. "Mechanically deposited tungsten disulfide saturable absorber for low-threshold Q-switched erbium-doped fiber laser." Applied Physics B 123, no. 8 (2017): 221.
- Hejazi, Davoud, Shuangjun Liu, Sarah Ostadabbas, and Swastik Kar. "Bayesian Inference-enabled Precise Optical Wavelength Estimation using Transition Metal Dichalcogenide Thin Films." arXiv preprint arXiv:1901.09452 (2019).
|
-
FAQ
1. What is the best method to dissolve/etch an AAO Template?
There are two methods that we recommend. Please make the best adjustments based on your own experiment.
Slow Removal: Depending on the microstructure evolution, a slow removal of AAO layer may be preferred. This can be achieved by using 5 wt% phosphate acid at 30º Celsius. Usually, this process is suitable for Single-Pass AAO.
Rapid removal: Use heated 3M NaOH or HCl solution, depending on your specific needs.
2. What's the thickness of the Al substrate?
The Al substrate is 150microns thick.
3. What is the best method for cutting an AAO Template?
The method we recommend involves using a metal ruler, a sharp knife or blade, a clean cloth (specialized cleanroom wipes suggested), and a hard flat surface (glass or steel).
Cutting Method:
1. Cover the hard surface with a cleanroom wipe or clean cloth.
2. Place the AAO sample on the wipe with the Al substrate (back side) facing up.
3. Align the metal ruler parallel to the cut you want to make.
4. Press down firmly on the ruler and slice the AAO sample several times along the edge of the ruler.
5. If the AAO sample is not cut through completely, slowly and carefully bend the template until it comes apart.
6. Finally, the AAO sample can be cleaned by ultrasonic cleaning <30 seconds using acetone, ethanol, isopropyl alcohol or deionized water.
Research Citations of ACS Material Products
- Jaworski, Nazariy, and Nazariy Andrushchak. “A method of nanoporous anodic aluminum oxide structure modeling based on Bezier curves generation.” 2017 14th International Conference The Experience of Designing and Application of CAD Systems in Microelectronics (CADSM), 2017, doi:10.1109/cadsm.2017.7916085.
- Fleming, Evan, Feng Du, Eric Ou, Liming Dai, and Li Shi. "Thermal conductivity of carbon nanotubes grown by catalyst-free chemical vapor deposition in nanopores." Carbon 145 (2019): 195-200.
- Fleming, Evan. "Multi-scale investigation of composite materials for enhanced phase change thermal storage systems." PhD diss., 2018.
- Jaworski, Nazariy, Marek Iwaniec, and Mykhaylo Lobur. "Implementation Features of Fibrous Composite Microlevel Structure Model Construction Based on Bezier Curves Generation and OpenCL Technology Usage." Machine Dynamics Research 41 (2017).
- Liu, Cong. "Nanoporous materials chemistry: From gas separation to high pressure chemistry." (2017).
|
-
FAQ
1. What is the rare earth metal used in the zeolitic framework?
It contains La2O3 and CeO2.
Research Citations of ACS Material Products
- Cha, Junyoung, Young Suk Jo, Hyangsoo Jeong, Jonghee Han, Suk Woo Nam, Kwang Ho Song, and Chang Won Yoon. "Ammonia as an efficient CO X-free hydrogen carrier: Fundamentals and feasibility analyses for fuel cell applications." Applied Energy 224 (2018): 194-204.
|
-
FAQ
1. Is there any difference between the two types in the gas adsorption process?
There is no difference in the gas adsorption process between the two types (Type A and Type B).
|
-
FAQ
1. What are the calcining conditions of ZSM-11?
ZSM-11 is calcined at about 500 degrees Celsius for 6 hours in the air.
|
-
FAQ
What is ZSM-22?
ZSM-22, an orthorhombic high silica zeolite (Cmcm, a = 13.86 ± 0.03Å, b = 17.41 ± 0.04Å, and c = 5.04 ± 0.02Å), has a framework consisting of 5-, 6- and 10-rings. The structure contains ferrierite sheets of the type previously found in ZSM-5, ZSM-11 and ZSM-35 and sheets of 6-rings similar to those of the rare zeolite bikitaite. The channel system is linear unidirectional and one-dimensional (noninterconnecting) with 10-membered ring openings which are 5.5 × 4.5 Å. The 10-ring channels are smaller than those found previously in ZSM-5, ZSM-11 and ZSM-35. [1]
[1] Kokotailo, G. T., et al. "The framework topology of ZSM-22: A high silica zeolite." Zeolites 5.6 (1985): 349-351.
Research Citations of ACS Material Products
- Zhou, Yuping, et al. “Selective conversion of castor oil derived ricinoleic acid methyl ester into jet fuel.” Green Chemistry, vol. 18, no. 19, 2016, pp. 5180–5189., doi:10.1039/c6gc00942e.
- Gołąbek, Kinga, et al. “Standard and rapid scan infrared spectroscopic studies of o-Xylene transformations in terms of pore arrangement of 10-Ring zeolites – 2D COS analysis.” Dalton Transactions, vol. 46, no. 30, 2017, pp. 9934–9950., doi:10.1039/c7dt00644f.
- Kim, Jaeheon, Seung Won Han, Jeong-Chul Kim, and Ryong Ryoo. "Supporting Nickel To Replace Platinum on Zeolite Nanosponges for Catalytic Hydroisomerization of n-Dodecane." ACS Catalysis 8, no. 11 (2018): 10545-10554.
- Bello Jurado, Estefanía. "ESTUDIO DE TRATAMIENTOS ÁCIDO/BÁSICOS EN ZEOLITAS MONODIMENSIONALES. CORRELACIÓN ENTRE PROPIEDADES TEXTURALES Y ACTIVIDAD CATALÍTICA." (2019).
|
|
-
FAQ
What is the maximum operating temperature, so that ZSM-5 Adsorbent Series are not damaged?
The maximum temperature is about 500℃ for ZSM-5 Adsorbent Series not to be damaged.
Research Citations of ACS Material Products
- Zhu, Bo, et al. “A method for defect repair of MFI-Type zeolite membranes by multivalent ion infiltration.” Microporous and Mesoporous Materials, vol. 237, 2017, pp. 140–150., doi:10.1016/j.micromeso.2016.09.011.
|
-
FAQ
What is the maximum operating temperature, so that ZSM-5 Catalyst are not damaged?
The maximum temperature is about 500℃ for ZSM-5 Catalayst not to be damaged.
Research Citations of ACS Material Products
- Yu, Joyleene Ruth. “Bio-Oil upgrading through biodiesel emulsification and catalytic vapour cracking.” Thesis / Dissertation ETD, 2014.
- Wei, Lin, and James Julson. Rotating Fluidized Bed Catalytic Pyrolysis Reactor.
- Mahadevan, Ravishankar, et al. “Physical and Chemical Properties and Accelerated Aging Test of Bio-Oil Produced from in Situ Catalytic Pyrolysis in a Bench-Scale Fluidized-Bed Reactor.” Energy & Fuels, vol. 29, no. 2, 2015, pp. 841–848., doi:10.1021/ef502353m.
- Chiaramonti, David, et al. “Bio-Hydrocarbons through catalytic pyrolysis of used cooking oils and fatty acids for sustainable jet and road fuel production.” Biomass and Bioenergy, vol. 95, 2016, pp. 424–435., doi:10.1016/j.biombioe.2016.05.035.
- Howe, Russell F., et al. “Application of Inelastic Neutron Scattering to the Methanol-to-Gasoline Reaction Over a ZSM-5 Catalyst.” Catalysis Letters, vol. 146, no. 7, 2016, pp. 1242–1248., doi:10.1007/s10562-016-1742-5.
- Rezaei-Dashtarzhandi, M., et al. “Zeolite ZSM5-Filled PVDF Hollow Fiber Mixed Matrix Membranes for Efficient Carbon Dioxide Removal via Membrane Contactor.” Industrial & Engineering Chemistry Research, vol. 55, no. 49, 2016, pp. 12632–12643., doi:10.1021/acs.iecr.6b03117.
- Kuhn, John N. , and Babu Joseph. Systems and methods for producing liquid hydrocarbon fuels.
- Zhu, Bo, et al. “Diffusion behaviour of multivalent ions at low pH through a MFI-Type zeolite membrane.” Desalination, 2017, doi:10.1016/j.desal.2017.09.033.
- Cha, Junyoung, Young Suk Jo, Hyangsoo Jeong, Jonghee Han, Suk Woo Nam, Kwang Ho Song, and Chang Won Yoon. "Ammonia as an efficient CO X-free hydrogen carrier: Fundamentals and feasibility analyses for fuel cell applications." Applied Energy 224 (2018): 194-204.
- Tshikesho, Redemptus S., Ajay Kumar, Raymond L. Huhnke, and Allen Apblett. "Catalytic Co-Pyrolysis of Red Cedar with Methane to Produce Upgraded Bio-oil." Bioresource Technology (2019).
|
-
FAQ
1. What the bulk density of Nano H-ZSM-5, Powder, Molar 52?
A: The bulk density of Nano H-ZSM-5, Powder, Molar 52 is 0.187g/mL.
2. What the bulk density of ZSM-5 Series Zeolite Powder, P-38?
A: The bulk density of ZSM-5 Series Zeolite Powder, P-38 is 0.356g/mL.
3. What is the morphology of ZSM-5 Series Zeolite Powder, P-38?
A: The morphology of ZSM-5 Series Zeolite Powder, P-38 is polycrystal. It is white powder.
Research Citations of ACS Material Products
- Chifiriuc, M. Carmen, et al. “Influence of hybrid inorganic/Organic mesoporous and nanostructured materials on the cephalosporins’ efficacy on different bacterial strains.” IET Nanobiotechnology, vol. 6, no. 4, Jan. 2012, pp. 156–161., doi:10.1049/iet-nbt.2011.0066.
- Zhu, Bo, et al. “Temperature and Pressure Effects of Desalination Using a MFI-Type Zeolite Membrane.” Membranes, vol. 3, no. 3, 2013, pp. 155–168., doi:10.3390/membranes3030155.
- Wagner, Jonathan L., et al. “Catalytic cracking of sterol-Rich yeast lipid.” Fuel, vol. 130, 2014, pp. 315–323., doi:10.1016/j.fuel.2014.04.048.
- Lazdovica, K., et al. “Catalytic pyrolysis of wheat bran for hydrocarbons production in the presence of zeolites and noble-Metals by using TGA-FTIR method.” Bioresource Technology, vol. 207, 2016, pp. 126–133., doi:10.1016/j.biortech.2016.01.117.
- Lazdovica, K., et al. “Comparative wheat straw catalytic pyrolysis in the presence of zeolites, Pt/C, and Pd/C by using TGA-FTIR method.” Fuel Processing Technology, vol. 138, 2015, pp. 645–653., doi:10.1016/j.fuproc.2015.07.005.
- Bianchini, Emma, et al. “Pyrolysis of spirulina and zeolite cracking over HZSM-5. An analytical investigation on the chemical route of bio-Oil from cultivation to combustion.” Journal of Analytical and Applied Pyrolysis, vol. 126, 2017, pp. 230–238., doi:10.1016/j.jaap.2017.06.004.
- Zhu, Bo, et al. “A method for defect repair of MFI-Type zeolite membranes by multivalent ion infiltration.” Microporous and Mesoporous Materials, vol. 237, 2017, pp. 140–150., doi:10.1016/j.micromeso.2016.09.011.
- Williamsona, Philip A., Pavithra M. Shanthib, Ramalinga Kurubac, Prashanth J. Hanumanthac, and Prashant N. Kumtaa. "The Effect of Zeolite Additives on Li-ion Conductivity of Gel-Polymer Electrolytes." Highlighting Undergraduate Research at the University of Pittsburgh Swanson School of Engineering (2018): 87.
- Muñoz-Olasagasti, M., A. Sañudo-Mena, J. A. Cecilia, M. López Granados, P. Maireles-Torres, and R. Mariscal. "Direct Conversion of Levulinic Acid into Valeric Biofuels Using Pd Supported Over Zeolites as Catalysts." Topics in Catalysis: 1-10.
- Schulzke, Tim, S. Conrad, B. Shumeiko, M. Auersvald, David Kubička, and L. F. J. M. Raymakers. "Fuels from Reliable Bio-based Refinery Intermediates: BioMates." Waste and Biomass Valorization (2019): 1-20.
- Shehab, Amal. "The Role of Carbon in the Catalytic Isomerisation-Cracking of n-Alkanes." PhD diss., University of Sheffield, 2018.
|